2 2.1 Neural Communication
Section Learning Objectives
Identify the electrical and chemical processes that allow neurons to communicate with each other.
Basic Chemistry and Physical Properties that are important in neural processing.
Neural signaling involves both chemical and electrical processes. To better understand neural communication, it is important to consider some chemical and physical properties.
There are four chemical elements that are important in neural signaling. You should be familiar with their symbols. They are:
- Sodium = Na+
- Potassium = K+
- Chloride = Cl–
- Calcium = Ca2+
The positive and negative part of the symbols represent that these are charged ions. They indicate that the atoms have lost (+) or gained (-) electrons, resulting in an imbalance to protons and electrons. This is important within a neuron as positive and negative charges attract each other, which is an important part of neuronal functioning.
The neural signals also have electrical properties. Electricity is a flow of electrons from a body that has a higher charge (more electrons) to an area that has a lower charge (fewer electrons). The difference in the voltage between the two sources is measured in volts and is referred to as an electrical potential. This is illustrated in Figure 2.1. Voltage can be defined as the force required to move the charges together. In the human body, the voltage changes we measure are at the level of microvolts (µV; 1 volt = 1,000,000 µV) for neural signals and millivolts (mV; 1 volt = 1,000 mV) for most muscle movements. These are much weaker signals than the voltage that we find in typical batteries (1.5 V for AA battery, Wikipedia) or powering our homes (120 V at 60 Hz, Wikipedia). The current is the rate at which the charges move (electrical charge/time). It is measured in amperes (amps).
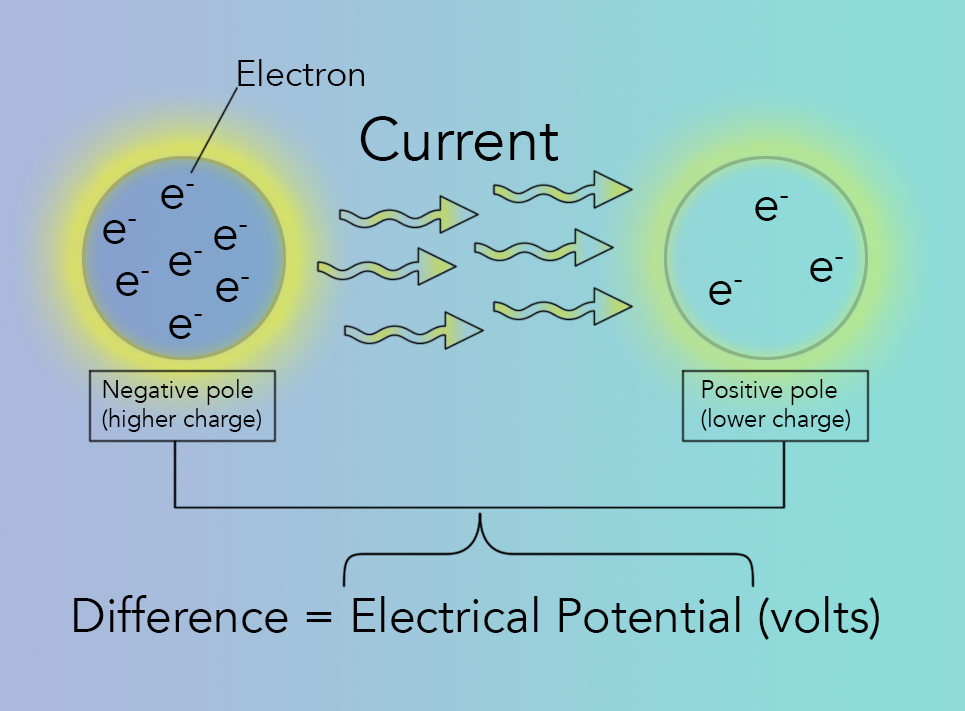
Recording Electrical Signals
Scientists have understood that the nervous system has electrical properties since the 1800s. Richard Canton first recording electrical signals from the human scalp. Around the same time others were applying electrical signals to the brain and watching how those signals elicited behaviors. in humans and animals. Today scientists can study electrical signals in the human body with many types of tools, such as the electroencephalogram (EEG).
Action Potentials
In Chapter 1 we learned the basic structure of the neuron, the cell body, the dendrites, and the axon. We learned that there is a synapse, a space, between neurons that a signal must cross to transmit information from one neuron to the next. In this section, we will learn about how that signal is transmitted with in a neuron. In the next section, we will learn about communication between neurons at the synapse.
An important aspect of neural communication is based on properties of the membrane of the neuron that allow for electrical and chemical signaling. The membrane separates in the intracellular fluid from the extracellular fluid. There are four important properties that maintain the resting potential of the neuron.
One aspect is the concentration gradient of sodium (Na+) and potassium (K+) ions. In the resting state, sodium ions are concentrated outside the cell, and potassium ions are concentrated inside the cell. Based on the principle of diffusion, ions move from areas of higher concentration to areas of lower concentration.
A second property is based on the voltage differences between the intracellular and extracellular fluids. These two fluids have different electrical charges, creating a membrane potential. The membrane potential of the neuron at rest is -70 mV inside the neuron relative to outside the neuron. The negative charge inside the cell reflects the presence of chloride (Cl-) and large proteins. The difference in charges across the membranes attract. The similar charges repel each other. This is related to the third quality of the membrane, selective permeability. While K+ ions can readily flow across the membrane through potassium pores, the channels for the Na+ ions are not open when the membrane is at rest.
The final quality of the resting potential, unlike the first three, requires energy on the part of the cell. This is the sodium-potassium pump. The pump brings in two K+ ions for every 3 Na+ ions it removes from the cell.
When a neuron receives signals from the dendrites that sum in the cell body to generate a signal, the channels for Na+ open on the axon, allowing the sodium ions to enter the cell due to the concentration gradient and the electrical potential difference. As the sodium enters the cell, the membrane potential increases, a process called depolarization. If the change in the charge increases, depolarizes, enough to reach the threshold of excitation, an action potential is begun.
As more pores open and more Na+ ions enter the cell, the membrane potential reaches its peak action potential at around +30 mV. Then the sodium channels begin to close and the potassium channels open. This results in the membrane potential decreasing, a process called repolarization. For a brief period the cell becomes more negative than usual, that is it is hyperpolarized. Then it returns back to a resting state. During the period of hyperpolarization, another action potential cannot be started. The action potential is very brief, lasting about 1 millisecond.
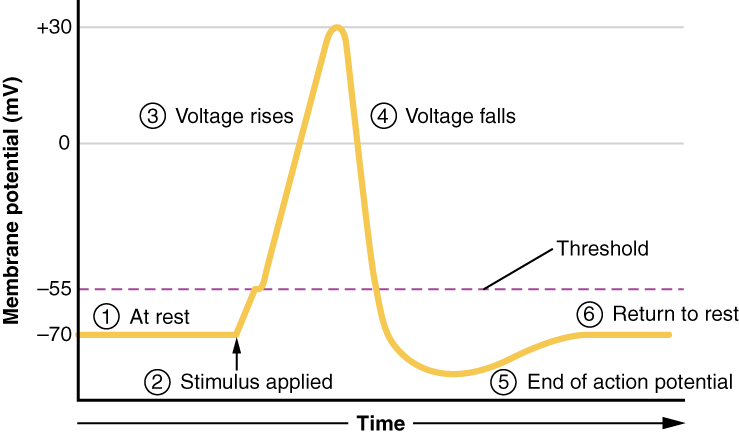
The electrical signal of the action potential moves down the axon from the cell body to the axon terminals. It only goes in one direction. The sodium channels open in sequence down the axon. Once it starts, it continues down to the axon terminal. This is an all-or-none phenomenon. If it starts it must continue. It has been compared to a gun firing or an email being sent – once triggered or sent, it cannot be undone. The action potential retains the same strength along the axon. It doesn’t fade out as it gets closer to the axon terminal. To get stronger signals, the potentials must come more quickly in succession. Weaker signals come at a slower rate.
Examples
- APA Online Psychology Library Demonstration of an Action Potential
- For a video review check out: Lights, camera, Action potential