5 Climate Change and Agriculture
Marjorie Jauregui
“Adaptation to climate change involves moving from the inevitable to the sustainable.”
—Galindo et al., 2015
Learning Objectives
At the end of this chapter, students will be able to:
- Explain why the climate is changing and the factors that are involved in the process.
- Understand how human actions are influencing global warming.
- Examine the connections among climate change, agriculture, and food security.
- Identify common mitigation strategies employed by farmers around the world.
Chapter Outline
Learning Objectives
Definitions
Introduction
- Causes of Climate Change
- Natural causes of climate change
- Anthropogenic climate change
- Effects of Climate Change on Agriculture
- Soil
- Crops
- Livestock
- Pests
- 2.1 Impacts of climate change on food security
- Adaptation and Mitigation Strategies
- Case Studies
- 4.1 – South America – Colombia – Coffee
- 4.2 –North America – United States – Corn and soybean
- Conclusion
- References
Definitions
- Weather: Variations in the state of the atmosphere at a given location that may occur at a particular time or over a short time (National Geographic, 2023).
- Climate: General weather conditions usually found in a particular place, or the long-term state of the atmosphere in a specific geographical area. Since weather is variable and can change over short periods of time, a region’s weather patterns, usually tracked for at least 30 years, are considered to define the climate (National Geographic, 2024).
- Anthropogenic climate change: Environmental change caused or influenced by people, whether directly or indirectly (U.S. Geological Survey, 2015).
- Greenhouse gases: Gases that trap heat in the atmosphere (U.S. Environmental Protection Agency, 2024) and are naturally part of the Earth’s atmosphere. They include carbon dioxide (CO2), methane (MH4), ozone, nitrous oxide (N2O), chlorofluorocarbons, and water vapor (NASA).
- Monoculture or mono-cropping: Agricultural practice of growing one crop species in a field at a time.
Introduction
Climate change refers to significant alterations in the long-term average of daily weather patterns in specific locations (Kilsby et al., 2007). As climatic conditions evolve, floods, water scarcity, heat waves, wildfires, droughts, and storms are becoming increasingly frequent and severe (Huber & Gulledge, 2011). Estimates suggest that over the past five decades, these extreme events have resulted in more than two million deaths and trillions of dollars in infrastructure damage, with over 70% of these losses attributed to climate-related factors (Clarke et al., 2022; Holzheu & Turner, 2018; Kron et al., 2012). While abrupt climate-related events are often attributed to natural causes, reports from the Intergovernmental Panel on Climate Change (IPCC) indicate otherwise. Since the initiation of systematic scientific assessments in the 1970s, it has become an established fact that human activities, starting in the mid-1800s, have significantly accelerated the warming of Earth’s climate (IPCC, 2023). Scientists assert that human-induced activities such as deforestation, fossil fuel production, and the degradation of terrestrial and marine ecosystems are driving warming temperatures and posing threats to biodiversity, agriculture, and food security (Harter et al., 2018; Papadimitriou, 2004; Ramieri et al., 2011).
Agriculture is highly dependent on environmental factors that influence crop yield and nutritional quality. Soil composition, water availability, terrain characteristics, and climate-related factors such as light, temperature, air, precipitation, relative humidity, and wind collectively can harm crop growth and yield; they can also affect the regional suitability of cultivated crops (Baker & Capel, 2011). Research suggests that climate change could lead to a reduction in global food availability, although its impact on crop yields is likely to vary by region. Some areas may experience negative effects while others may benefit, depending on the severity of these changes (Bigolin & Talamini, 2024). For instance, a study published in 2021 revealed that staple crops like corn and soybean in the United States could see yield reductions of 41% and 87%, respectively, due to inadequate adaptation to shifting environmental conditions. The authors projected further declines in average yields by 2050 (Yu et al., 2021). Conversely, a 2024 literature review showed that while many studies predict an approximate 80% reduction in corn yield in Brazil due to rising temperatures under climate change scenarios, several others anticipate up to a 60% increase in soybean yield due to higher atmospheric CO2 in the same region (Bigolin & Talamini, 2024).
As observed, agriculture is acutely sensitive to shifts in weather and climate patterns, making any sudden changes or intensifications in climate events like high temperatures, droughts, and floods particularly impactful. Moreover, these extreme weather shifts contribute to the proliferation of pests, weeds, and fungi that thrive in CO2-rich environments; warmer temperatures; and wetter climates (Environmental Protection Agency [EPA], 2017). These compounding factors present significant challenges for farmers all around the world.
Farmers are among the most vulnerable to climate change. Negative weather conditions leading to decreased agricultural productivity have forced many of them to reassess the source of their livelihoods. Since food production is their primary source of income, declines in output significantly affect their quality of life. For farmers and their families, food has become increasingly scarce and expensive (Devendra, 2012). Fortunately, increased knowledge and access to more sustainable technologies have enhanced the resilience of many farmers to climate change. Although extreme climate events cannot always be predicted and prevented, more farmers have developed mitigation strategies. Collaborating within their communities, they work toward enriching their soil with nutrients and making their crops more resilient against biotic (i.e., factors related to living organisms such as viruses, bacteria, and fungi) and abiotic (i.e., nonliving factors like extreme temperatures, salinity, drought, flooding, and nutrient deficiencies) threats. This concerted effort aims to contribute to food security sustainability despite the challenges presented by climate change.
Causes of Climate Change
Natural Causes of Climate Change
While human activities are often recognized as the main driver of warming temperatures, it is important to acknowledge the role of natural phenomena in changes in the global climate. Before the Industrial Revolution, variations in climate were influenced by factors such as solar variability, volcanic eruptions, and tectonic plate movements. Data collected over 150–200 years from meteorological stations on Earth’s surface and orbiting satellites allow scientists to reconstruct historical temperatures and analyze climate models. Solar variability, including changes in Earth’s orbit and solar radiation, played a significant role in climate fluctuations between 1750 and 1950 (National Aeronautics and Space Administration [NASA], 2010). Additionally, volcanic eruptions release sulfur dioxide into the atmosphere, forming aerosols that reflect sunlight and temporarily cool the planet. These natural events, while impactful, have historically produced short-term effects on global temperatures lasting from two to several years, depending on the magnitude of the event.
However, the frequency and magnitude of these natural phenomena pale in comparison to the rapid global warming observed in recent decades. Human-induced greenhouse gas (GHG) emissions dating back to the post–Industrial Revolution era have overwhelmed the natural climate fluctuations. While natural factors play a role, scientific evidence supports the conclusion that human activities are the primary driver of the abrupt climate change that is influencing the warming of the planet (IPCC, 2022; NASA, 2010).
Anthropogenic Climate Change
According to the IPCC’s Sixth Assessment Report (2022), there is an agreement among scientists that human activity is the main contributor to climate change, with industrial activities being the primary cause of severe environmental damage. In the report, the experts concluded that the main drivers of anthropogenic climate change are land use, aerosols, and GHG emissions. In the atmosphere, GHGs such as carbon dioxide (CO2), methane (CH4), and nitrous oxide (N2O) function like glass panels in a greenhouse. They absorb the heat from the sun, much like a glass panel traps warmth within a greenhouse. Once absorbed, these gases trap the heat in the atmosphere and block it from escaping into space (Sparks, 2020). This controlled retention and the release of heat contribute to a warming effect crucial for sustaining life on Earth, mirroring the functions of a greenhouse in nurturing plant growth. While these greenhouse gases exist naturally in a balanced state within the atmosphere, human activities significantly contribute to their increased accumulation through emissions from industrial activities. As this accumulation grows, the atmosphere traps more heat than necessary, leading to global warming and subsequent climate changes (IPCC, 2014). According to the IPCC (2014), over the last three decades, GHG emissions have led to a rise in Earth’s surface temperature by 0.6°C.
The issue of greenhouse gases and their impact on Earth’s climate has been acknowledged for many years. As shown in Table 1, since the pre-industrial times, concentrations of the greenhouse gases N2O, CO2, and CH4 in the atmosphere have increased by 18%, 47%, and 60%, respectively (EPA, 2023), although some sources indicate a 160% increase in CH4 from the 1700s to 2020 (Jeffry et al., 2021; Loulergue et al., 2008). These increases are primarily driven by global population growth and economic expansion, which have led to a greater demand for fossil fuels to generate power for industries. As a result, the increased use of fossil fuels has become one of the major contributors to climate change (Tans, 2009). In addition, the GHG emissions caused by extensive deforestation for industrial and agricultural expansion are leading to an irreversible buildup of greenhouse gases in the atmosphere. With fewer trees available to absorb CO2 and produce oxygen, the natural recycling process is disrupted, leading to the accumulation of more CO2 in the atmosphere (Mbow et al., 2017).
Greenhouse gas |
Pre-industrial concentration (1700s) |
2021 concentration |
Increase (%) |
Carbon dioxide (CO2) |
280 ppm |
414 ppm |
48 |
Methane (CH4) |
~ 900 ppb |
1800 ppb |
50–60 |
Nitrous oxide (N2O) ** |
280 ppb |
334 ppb |
19 |
Source: EPA (2023)
Ppm = parts per million, ppb = parts per billion.
** N2O concentrations have risen since the 1920s
Effects of Climate Change on Agriculture
What we know:
- The climate is changing.
- Agricultural productivity is highly influenced by climate conditions.
- Because agriculture is vital for the development and well-being of life on Earth, climate change has emerged as a global concern.
Soil
Climate change-induced alterations, such as an increased frequency of drought and floods, significantly affect the foundation of agriculture: soil (Corwin, 2021; Kalra et al., 2007). Soil quality, crucial for agricultural productivity, depends on different factors. One of the most important is the microbial communities. These communities are intricately linked to the balance of GHG concentrations and temperature levels. Climate change disrupts soil microbial communities by altering nutrient availability, thereby reshaping their composition and functionality (Jansson & Hofmockel, 2020). For instance, elevated temperatures may promote the proliferation of certain microbial species, leading to their dominance and restructuring of the community. Moreover, prolonged periods of intense rainfall, wind, and drought exacerbate soil erosion, diminishing soil fertility and directly affecting crop yields and quality (Kalra et al., 2007).
Crops
Crops are highly sensitive to climatic conditions, with persistent alterations in climate gradually affecting the soil quality, the main source of nutrients for crops. This leads to significant changes in soil composition, affecting crops’ adaptation abilities, phenology, morphology, and nutritional value and ultimately impacting their productivity yields (Adams et al., 1998; Murungu et al., 2011). Climate-related events such as heat waves pose a threat to crop yields by inducing severe droughts that take up soil moisture through evaporation, making it difficult for plants to access the water and nutrients necessary for their adequate development and functioning. Moreover, increased atmospheric CO2 concentrations accelerate plant photosynthesis and growth, reducing plants’ water intake and their levels of nitrogen, protein, minerals, and vitamins (Mbow et al., 2019; Taub, 2010).
In addition, in modern agriculture, technological change and public policy have influenced farmers to work toward industrialized and monoculture, or mono-cropping, farming practices (Wallinga, 2009). While these methods can be efficient, they make farmers more vulnerable to abrupt variations in climate. When a monoculture farm (a farm that produces only one crop or product) is affected, the entire system is at risk of failure, resulting in substantial losses for farmers (Bourke et al., 2021). On the other hand, the diversification of crops not only serves as an economic strategy but also contributes to enhancing productivity. Crop rotation is a highly sustainable agricultural practice. It contributes to soil fertility by balancing nutrient management, prevents pest proliferation, disrupts pathogen reproduction, and helps to increase crop yields (Shah et al., 2021). Ultimately, this approach has significant benefits for food security.
Livestock
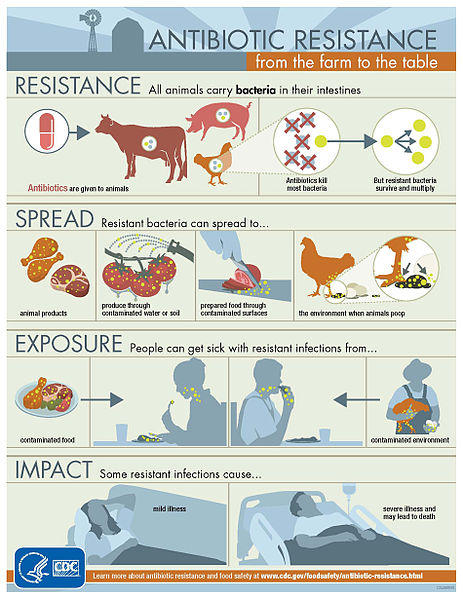
Long Description
The infographic titled “Antibiotic Resistance from the Farm to the Table” illustrates the pathway of antibiotic resistance. It is divided into four sections: Resistance, Spread, Exposure, and Impact.
- In the “Resistance” section, the image depicts animals such as a cow, pig, and chicken, showing how they carry bacteria in their intestines. A pill icon represents antibiotics given to animals, which kill most bacteria but allow resistant bacteria to survive and multiply. Illustrated arrows indicate the direction of the spread.
- The “Spread” section shows how resistant bacteria can contaminate animal products, produce through water or soil, prepared food, and the environment when animals defecate.
- In the “Exposure” section, it shows individuals exposed by consuming contaminated food or being in contaminated environments, depicted through images of a person eating and coughing. Illustrated arrows indicate the direction of the spread.
- The “Impact” section illustrates outcomes of some resistant infections, showcasing a person in bed with mild illness and another with severe illness leading to death.
At the bottom, there is a link for more information.
Transcribed Text:
Antibiotic Resistance from the farm to the table. Resistance: All animals carry bacteria in their intestines. Antibiotics are given to animals. Antibiotics kill most bacteria. But resistant bacteria survive and multiply. Spread: Resistant bacteria can spread to animal products, produce through contaminated water or soil, prepared food through contaminated surfaces, the environment when animals poop. Exposure: People can get sick with resistant infections from contaminated food, contaminated environment. Impact: Some resistant infections cause mild illness, severe illness and may lead to death. Learn more about antibiotic resistance and food safety at www.cdc.gov/foodsafety/antibiotic-resistance.html.
This is a story of two contrasting perspectives. On one hand, raising animals for consumption involves the waste of many natural resources. Livestock farming utilizes and contaminates vast amounts of water and contributes significantly to deforestation. In addition, at least 17% of GHG emissions originate from livestock, largely due to methane production (Carr et al., 2023). One example of the negative consequences of raising animals for food production is found in the Brazilian Amazon. Seventy-five percent of deforestation is attributed to livestock agriculture (Millstein, 2022). Landowners and farmers cut down the trees and use the wood to build ranches for their animals and create open land to cultivate maize and soy to feed their livestock. But climate change also threatens the animals living on these farms. Imagine the conditions on an industrial farm, where animals live in tight spaces. The rise in temperature in confined spaces provokes metabolic alterations and immune suppression in animals, making them more vulnerable to diseases (Millstein, 2022). In addition, the limited space between animals creates the right environment for the rapid spread of diseases, which leads to an increase in the rate of animal death (Baumgard et al., 2012; Bett et al., 2017). The Centers for Disease Control and Prevention (CDC) has been emphatic in reporting the link between disease presence in farm animals and the safety of products destined for human consumption (Fig. 1). Bacteria living in animal intestines can develop resistance to antibiotics and continue proliferating. These resistant bacteria can propagate and spread disease to other animals, contaminating meat and other animal products. Consequently, people who handle or eat these products are at risk of exposure and may develop severe infections that can lead to fatal outcomes.
Pests
Unlike crops, animals, and humans, pests are very adaptable organisms. While we see extreme weather events as a threat, agricultural pests see these events as opportunities to live and expand. For example, the increase in temperature can increase the overwintering survival of insects, the number of generations, and the risk of more invasive species (Skendžić et al., 2021).
The effects of climate change, such as rising temperatures and humidity levels, in addition to the weakening of natural predators, create favorable conditions not only for insects, but also for fungal, and virus proliferation, leading to increased infections and health issues for both plants and animals (Viguera et al., 2017). The development of disease due to the infection of pests can cause significant losses that may ultimately lead to starvation of certain populations, especially in developing countries. According to Savary et al. (2019), it has been estimated that between 10 and 28% of global food production is lost to pests. Pest infestation on farms limits agricultural output and places economic burdens on farmers. To combat these challenges, farmers often resort to investing in pesticides and other resources, further straining their finances (Shukla et al., 2021).
Example
A classic example of the harm caused by pests to a crop can be seen in 1840s Ireland. The fungus Phytophthora infestants (P. infestants), also known as “potato late blight fungus,” caused what we now know as the Irish Potato Famine. The disease spread rapidly among the potato crop, which was the main staple crop for the Irish population. The infestation resulted in the loss of half of the potato crop in 1845 and more than three-quarters of the total crop over the next seven years. Due to the limited knowledge and resources among farmers at that time, this infestation had a catastrophic impact on the Irish population. When the nightmare ended in 1852, the Famine registered one million Irish people dead with another million forced to leave their homes as refugees.
For a more detailed example, visit Section 4.1.
Impacts on Food Security
In earlier sections of this chapter, we explored the susceptibility of agriculture to climate change and the negative impact of unpredictable and extreme weather patterns on global food security. Analogous to a chain, food security relies on soil, crop and livestock quality, pest control, and farmer education. Each of these represents a link, and the integrity and strength of this chain depends on each of these links. Unfortunately, climate change is gradually breaking down the chain of global food security.
The impacts of climate change extend far beyond agricultural productivity. Fluctuations in temperatures and rainfall patterns disrupt crop growth and compromise crops’ nutritional quality, yielding a final product that fails to meet the energy and nutritional requirements of either humans or animals (Porter & Semenov, 2005; Sekaran et al., 2021). Agricultural losses make farming an unprofitable business, triggering a chain reaction that affects consumers: consumers witness an increase in global food prices that over time may cause them to modify their dietary habits (Viguera et al., 2017).
As this discussion suggests, climate change is changing the way we grow our food and the type of food we grow. For instance, some studies suggest that by 2050, because of the consequences of climate change, developing countries may experience a 7% reduction in the consumption of staple crops like wheat, rice, and corn that are essential for most of the global population (Nelson et al., 2009). As yield decreases, the staple crops will become scarce, affecting people’s access to affordable and nutritious alternatives and exacerbating food insecurity.
Unfortunately, factors such as geographic and economic limitations and a lack of knowledge or appropriate technology preclude farmers in developing countries from addressing the challenges of climate change. In addition, due to the lack of affordable food, populations living in poverty experience the devastating consequences of starvation and malnutrition (Phalkey et al., 2015). A clear example of this is the Irish Potato Famine (Section 2d).
Adaptation and Mitigation Strategies
Mitigation involves efforts to reduce the magnitude of change. For example, actions like using more types of renewable energies and reducing deforestation are measures of mitigation. Adaptation refers to the ability to adjust and minimize harmful effects. Examples include educating farmers, diversifying crops, and managing water resources more efficiently (Abbass et al., 2022).
According to Vijaya Ventaja Raman et al. (2012), achieving sustainable outcomes requires a balanced approach that integrates mitigation and adaptation strategies to face the challenges of climate change effectively. As humans, we have demonstrated that we can find our way into a more sustainable world. The reintroduction of ancestral agricultural practices together with technological advancements such as refined machinery and biotechnology have helped us and will continue helping us to develop more resilient crops.
Some successful strategies that farmers have been implementing in order to reduce the consequences of climate change are as follows:
- Large-scale afforestation, in other words, planting more trees. Planting trees can help to cool the environment. Through photosynthesis, plants intake one of the major greenhouse gases present in the atmosphere, CO2, transforming and releasing it in the form of O2 (Bala, 2014).
- Annual crop rotation. This practice helps with maintaining soil fertility and controlling pests, diseases, and weeds, in addition to improving agricultural efficiency (Volsi et al., 2022). (For more information, review Sections 2b and 4.2.)
- No till or reduced till. In this practice, seeds are sown directly into undisturbed soil to minimize soil eruption and increase soil carbon levels and biological activity (Cooper et al., 2021; U.S. Department of Agriculture [USDA, n.d.].
- Genetically modified organisms (GMOs). Advancements in biotechnology have been used to develop genetically climate- and pest-resilient crops. Although the practice is controversial, it has been proposed as an adaptation strategy to increase crop yield even during extreme weather events. One of the most famous examples of GMOs and climate adaptation is the genetically modified drought-tolerant corn. After its efficacy was demonstrated, the corn was approved for commercialization in the U.S. market (McFadden et al., 2019). (For more information about GMOs, review “Use of Genetically Modified Organisms in Global Agriculture.”)
Visit these links to learn more about farmers’ strategies for mitigating the effects of climate change:
Case Studies
South America – Colombia – Coffee
Colombia possesses a remarkable diversity of climates across its regions, an attribute that has helped it to become one of the major coffee producers in South America and to earn the appellation of “País Cafetero” (coffee country). Coffee plantations can be found in various parts of Colombia, with major production areas including the departments of Antioquia, Bolivar, Caldas, Cauca, Huila, Quindio, and Risaralda. However, it is the Coffee Triangle, involving the departments of Caldas, Quindio, and Risaralda, that often captures initial attention and where many farmers start. The principal cities of these departments are Manizales, Pereira, and Armenia (Fig. 2).
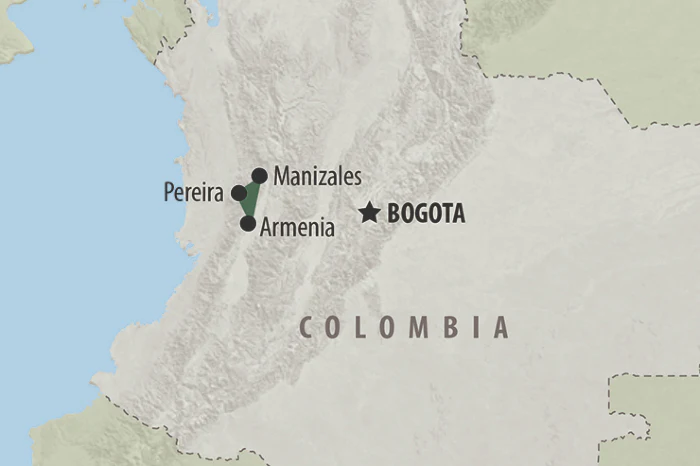
The Coffee Triangle, called in Spanish “Eje Cafetero,” has long been known for its optimal environmental conditions for agriculture. Located in the Andes, it is characterized by a perfect mixture of rainfall, atmospheric pressure, altitude, and mild temperatures for coffee farming. The presence of volcanoes enriches the soil of these farms with essential nutrients and minerals to promote the growth of coffee plants. Coffee farms hold deep-rooted historical and traditional significance for many families in Colombia, symbolizing a legacy of sharing and collaboration among farm families. Land ownership is traditionally divided among these families, with agricultural traditions passed down through the generations. However, this rich tradition faces significant challenges, and climate change is among the most pressing. Unpredictable weather patterns are increasingly disrupting coffee production on small farms. Coffee plants thrive in cooler climates, yet the most productive zones have experienced a rise in temperatures of 2 to 3°C, resulting in less favorable conditions for coffee cultivation. In addition, shifting weather patterns are exacerbating the risk of climate-related events such as floods and droughts across various Colombian regions, creating optimal environments for pests and the development and spread of diseases among plants (Fig. 3).
Although leaving behind their family lands and traditions is a hard decision, farmers have overcome these challenges by adopting three primary strategies: spraying pesticides, relocating coffee farms to different areas, and transitioning to other crops. However, these strategies are not without their own problems. Pesticide use poses water contamination concerns, while relocating farms is costly and risky. Although the transition to other crops could be also considered an expensive and risky solution, the option to cultivate more climate-resilient crops like pineapple and cassava appears to be a promising alternative that some farmers have successfully embraced.
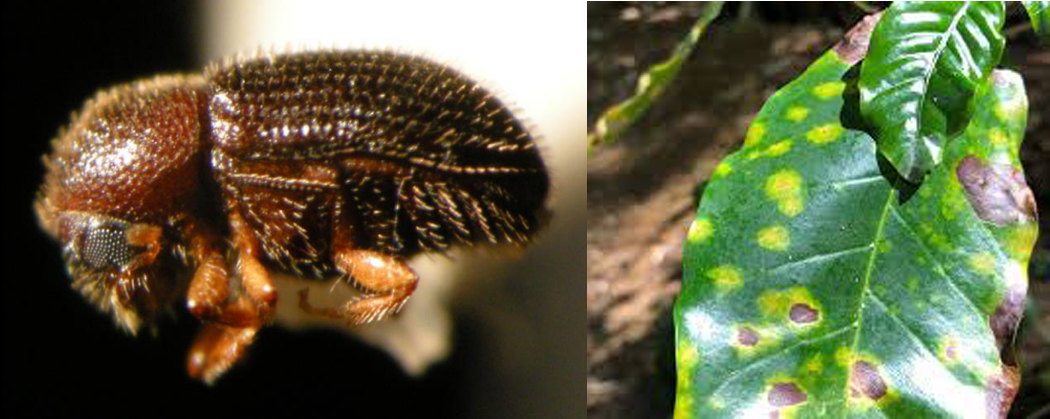
For more information, please visit the following 2 YouTube videos:
- The Colombian Coffee Triangle – The Past and Future of Colombia’s Most Famous Coffee Producing Region
- TWO DEGREES UP – PART ONE: COLOMBIA
North America – United States – Corn and soybean
The link between the Jones family and farming was established in the 1940s when the family settled on the land that is now known as the Jones Farm, located in Burnside, Illinois. Their agricultural activities started with a full-year crop rotation, which included growing maize, soybeans, alfalfa, and hay. Along with planting crops, the family kept a variety of animals, from beef and dairy cattle to pigs and poultry, resulting in the production of a wide range of food products.
“Being a farmer involves a lot of decision-making. Diversification is not always the best option when prioritizing crops,” said Kenneth (Ken) Jones, son of the first owner of the Jones Farm. Over the decades, gradual improvements have been made and are reflected in the family’s current farming operations. For instance, they stopped raising chickens and selling eggs and eventually ceased raising pigs and cattle as well. This evolution was influenced by changing climatic conditions. As temperatures in the Midwest rose, planting seasons came to start earlier in the year, demanding more labor.
Reflecting on climate change, Ken remarked, “There is no doubt that the climate is changing, but it has always changed.” He recalls a severe drought that affected the country when he was a child. He remembers his dad telling him about how the water scarcity and the extremely high temperatures harmed their agricultural harvests. Those were challenging times for farmers, particularly because they did not have the technology or the ability to restore productivity by sowing their fields in half a day as they do now. However, he recognizes that at that time, those events were isolated and changes to the climate were slow and gradual. Nowadays, weather changes are becoming more frequent and severe, with variations in droughts, flooding, and the occurrence of pests. According to Ken, these weather pattern alterations have a greater impact on farmers than landowners, affecting both their productivity and income.
Ken recognizes the farm’s resilience. Over the years, they have implemented several strategies to mitigate climate-related risks. For example, the Jones Farm has adopted crop rotation, an ancient agricultural practice that even today is crucial for preventing pest infestations and preserving soil health rotation between corn and soybean production year after year. Additionally, Ken emphasizes the significance of technological advancements such as GPS-guided systems and seed technology in enhancing agricultural efficiency. The Jones family’s harvest has expanded from 80–160 acres to 1,500–2,000 acres thanks to continuous developments in farming equipment and seed technology that have enabled the family to cultivate more land.
Ken anticipates that the accelerated change in weather patterns might lead to shifts in agricultural productivity in regions across the country. However, he thinks that technological advancements offer farmers hope for resilience, with more farmers adopting practices like not tilling land to optimize the crop yields of their farms.
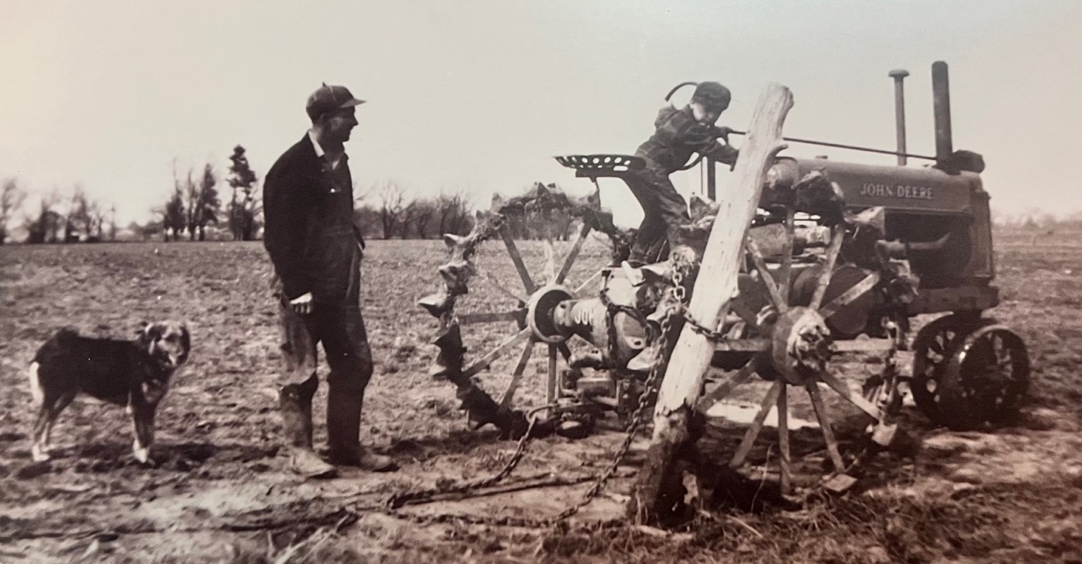
As for the future, Ken remains optimistic, recognizing his farm’s resilience and adaptability to any challenge that climate change can bring.
Conclusion
Efficient agricultural production is the result of the interaction of two factors: healthy soil and high-quality crops. When the two factors involved in this equation are deficient—meaning neither soil nor crops are high quality–the results are inadequate food availability and compromised food security. This leads to food products that fail to meet the energy and nutritional needs of either humans or animals.
As reviewed in this chapter, climate change is the main driver of the failure of the food production system. Climate change is a natural phenomenon that has been happening for many years. However, human activities have helped to intensify the frequency and severity of climate-related events.
Without immediate action, the devastating impacts of climate change will only worsen, producing a faster decline in global food availability. This, in turn, will increase the spread of diseases, posing an even greater threat to the global population’s health. For this reason, it is important for farmers to understand the drivers of climate change and implement adaptation and mitigation measures, and for all to continue to work toward building a more sustainable world for this generation and the many more to follow.
Further Exploration
Scientific articles and book chapters
- Altieri, M. A., Nicholls, C. I., Henao, A., & Lana, M. A. (2015). Agroecology and the design of climate change-resilient farming systems. Agronomy for sustainable development, 35(3), 869-890.
- Dang, H. L., Li, E., Nuberg, I., & Bruwer, J. (2019). Factors influencing the adaptation of farmers in response to climate change: A review. Climate and Development, 11(9), 765-774.
- Mozaffari, G. A. (2022). Climate change and its consequences in agriculture. The Nature, Causes, Effects and Mitigation of Climate Change on the Environment, 83.
- Nelson, G. C., Rosegrant, M. W., Koo, J., Robertson, R., Sulser, T., Zhu, T., … & Lee, D. (2009). Climate change: Impact on agriculture and costs of adaptation (Vol. 21). Intl Food Policy Res Inst.
Documentaries
- Floods, heavy rain, extreme weather – Why we need to build differently | DW Documentary YouTube video 1
- Changing Paradigms | Regenerative Agriculture: A Solution to our Global Crisis? | Tom’s Outdoors YouTube video 2
Check Your Knowledge!
- What are the main contributors to the GHG?
- What are the downsides of the implementation of monoculture farming?
- What is the relationship between climate change and the development of diseases in livestock?
- What are the two main reasons for farmers’ relocation in Case Study 1?
References
Abbass, K., Qasim, M. Z., Song, H., Murshed, M., Mahmood, H., & Younis, I. (2022). A review of the global climate change impacts, adaptation, and sustainable mitigation measures. Environmental Science and Pollution Research, 29(28), 42539–42559. Review of global climate change impacts, adaptation, and sustainable mitigation measures.
Adams, R. M., Hurd, B. H., Lenhart, S., & Leary, N. (1998). Effects of global climate change on agriculture: an interpretative review. Climate Research, 11(1), 19-30. Effects of global climate change on agriculture: interpretative review.
Bett, B., Kiunga, P., Gachohi, J., Sindato, C., Mbotha, D., Robinson, T., & Grace, D. (2017). Effects of climate change on the occurrence and distribution of livestock diseases. Preventive Veterinary Medicine, 137(B), 119–129. Effects of climate change on livestock diseases.
Bourke, P. M., Evers, J. B., Bijma, P., van Apeldoorn, D. F., Smulders, M. J., Kuyper, T. W., … & Bonnema, G. (2021). Breeding beyond monoculture: Putting the “intercrop” into crops. Frontiers in Plant Science, 12, 734167. Breeding beyond monoculture: Putting the intercrop into crops.
Carr, E. R., Diro, R., Naeve, K., Hamel, R., Beggs, M., Benson, C., Caldwell, B., Mbevi, L., Hall, T., Zook, D., Aldredge, H., Liming, K., Allognon, L., Crocker, T., & Mukupa N. (2023). Operationalizing USAID’s climate strategy to achieve transformative adaptation and mitigation in agricultural and food systems. Tetra Tech and the Board for International Food and Agricultural Development. USAID’s climate strategy for adaptation and mitigation in agriculture.
Clarke, B., Otto, F., Stuart-Smith, R., & Harrington, L. (2022). Extreme weather impacts of climate change: an attribution perspective. Environmental Research: Climate, 1(1), 012001. Extreme weather impacts of climate change.
Cooper, H. V., Sjögersten, S., Lark, R. M., & Mooney, S. J. (2021). To till or not to till in a temperate ecosystem? Implications for climate change mitigation. Environmental Research Letters, 16(5), Article 054022. To till or not to till: Climate change mitigation.
Corwin, D. L. (2021). Climate change impacts on soil salinity in agricultural areas. European Journal of Soil Science, 72(2), 842-862. Climate change impacts on soil salinity.
Galindo, L. M., Samaniego, J., Alatorre, J. E., Carbonell, J. F., Reyes, O., & Sánchez, L. (2015). Ocho tesis sobre el cambio climático y el desarrollo sostenible en América Latina (CEC/14/001). EUROCLIMA Climate Change Programme, Socioeconomic Component. Ocho tesis sobre el cambio climático y desarrollo sostenible en América Latina.
Hartter, J., Hamilton, L. C., Boag, A. E., Stevens, F. R., Ducey, M. J., Christoffersen, N. D., & Palace, M. W. (2018). Does it matter if people think climate change is human caused? Climate Services, 10, 53–62. Does it matter if people think climate change is human caused?.
Holzheu, T., & Turner, G. (2018). The natural catastrophe protection gap: Measurement, root causes and ways of addressing underinsurance for extreme events. Geneva Papers on Risk and Insurance—Issues and Practice, 43(1), 37–71. The natural catastrophe protection gap.
Intergovernmental Panel on Climate Change. (2014). Climate change 2014: Synthesis report. Contribution of working groups I, II and III to the Fifth Assessment Report of the Intergovernmental Panel on Climate Change. Core Writing Team: R. K. Pachauri and L. A. Meyer (Eds.). Climate change 2014: Synthesis report.
———. (2022). Climate change 2022: Impacts, adaptation and vulnerability. Working group II contribution to the Sixth Assessment Report of the Intergovernmental Panel on Climate Change. Lead Editors: H.-O. Pörtner and D. C. Roberts. Climate change 2022: Impacts, adaptation and vulnerability.
———. (2023). Contribution of working groups I, II and III to the Sixth Assessment Report of the Intergovernmental Panel on Climate Change. Core Writing Team: H. Lee and J. Romero (Eds.). Sixth Assessment Report: Synthesis report.
Jansson, J. K., & Hofmockel, K. S. (2020). Soil microbiomes and climate change. Nature Reviews Microbiology, 18(1), 35-46. Soil microbiomes and climate change.
Jeffry, L., Ong, M. Y., Nomanbhay, S., Mofijur, M., Mubashir, M., & Show, P. L. (2021). Greenhouse gases utilization: A review. Fuel, 301, Article 121017. Greenhouse gases utilization: A review.
Kalra, N., Chander, S., Pathak, H., Aggarwal, P. K., Gupta, N. C., Sehgal, M., & Chakraborty, D. (2007). Impacts of climate change on agriculture. Outlook on AGRICULTURE, 36(2), 109-118. Impacts of climate change on agriculture.
Kilsby, C. G., Jones, P. D., Burton, A., Ford, A. C., Fowler, H. J., Harpham, C., & Wilby, R. L. (2007). A daily weather generator for use in climate change studies. Environmental Modelling & Software, 22(12), 1705–1719. A daily weather generator for climate change studies.
Kron, W., Steuer, M., Löw, P., & Wirtz, A. (2012). How to deal properly with a natural catastrophe database: Analysis of flood losses. Natural Hazards and Earth System Sciences, 12(3), 535–550. Analysis of flood losses in natural catastrophe databases.
Loulergue, L., Schilt, A., Spahni, R., Masson-Delmotte, V., Blunier, T., Lemieux, B., Barnola, J-M., Raynaud, D., Stocker, T. F., & Chappellaz, J. (2008). Orbital and millennial-scale features of atmospheric CH4 over the past 800,000 years. Nature, 453, 383–386. Orbital and millennial-scale features of atmospheric CH4.
Mbow, H. O. P., Reisinger, A., Canadell, J., & O’Brien, P. (2017). Special report on climate change, desertification, land degradation, sustainable land management, food security, and greenhouse gas fluxes in terrestrial ecosystems (SR2). Paris: IPCC. Chapter 3: Desertification. Special report on climate change: Desertification chapter.
Mbow, C., Rosenzweig, C., Barioni, L.G., Benton, T.G., Herrero, M., Krishnapillai, M., Liwenga, E., Pradhan, P., Rivera-Ferre, M. G., Sapkota, T., Tubiello, F. N., & Xu, Y. (2019). Food security. In P. R. Shukla et al. (Eds.), Climate change and land (pp. 437–550). Climate change and land: Food security.
McFadden, J., Smith, D., Wechsler, S., & Wallander, S. (2019). Development, adoption, and management of drought-tolerant corn in the United States. Development and adoption of drought-tolerant corn in the U.S..
Millstein, S. (2024, July 24). How does livestock farming affect climate change? Sentient. How does livestock farming affect climate change?.
Murungu, F. S., Chiduza, C., Muchaonyerwa, P., & Mnkeni, P. N. S. (2011). Mulch effects on soil moisture and nitrogen, weed growth and irrigated maize productivity in a warm-temperate climate of South Africa. Soil and Tillage Research, 112(1), 58-65. Mulch effects on soil moisture and maize productivity.
National Geographic. (2023). Weather. Last updated October 19, 2023. National Geographic: Weather.
———. (2024). All about climate. Last updated January 2, 2024. All about climate.
National Aeronautics and Space Administration. (2010, June 3). Is current warming natural? Earth Observatory. Is current warming natural?.
———. (2024). What is the greenhouse effect? Last updated January 30, 2024. What is the greenhouse effect?.
Phalkey, R. K., Aranda-Jan, C., Marx, S., Höfle, B., & Sauerborn, R. (2015). Systematic review of current efforts to quantify the impacts of climate change on undernutrition. Proceedings of the National Academy of Sciences, 112(33), E4522-E4529.
Papadimitriou, V. (2004). Prospective primary teachers’ understanding of climate change, greenhouse effect, and ozone layer depletion. Journal of Science Education and Technology, 13(2), 299–307.
Porter, J. R., & Semenov, M. A. (2005). Crop responses to climatic variation. Philosophical Transactions of the Royal Society B: Biological Sciences, 360(1463), 2021-2035.
Ramieri, E., Hartley, A., Barbanti, A., Santos, F. D., Gomes, A., Hilden, M., Laihonen, P., Marinova, N., & Santini, M. (2011). Methods for assessing coastal vulnerability to climate change (ETC CCA Technical Paper 1/2011). European Topic Centre on Climate Change Impacts, Vulnerability and Adaptation, part of the European Environment Agency.
Savary, S., Willocquet, L., Pethybridge, S.J., Esker, P., McRoberts, N., & Nelson, A. (2019). The global burden of pathogens and pests on major food crops. Nature Ecology & Evolution, 3(3), 430–439.
Sekaran, U., Lai, L., Ussiri, D. A., Kumar, S., & Clay, S. (2021). Role of integrated crop-livestock systems in improving agriculture production and addressing food security–A review. Journal of Agriculture and Food Research, 5, 100190.
Shah, K. K., Modi, B., Pandey, H. P., Subedi, A., Aryal, G., Pandey, M., & Shrestha, J. (2021). Diversified crop rotation: An approach for sustainable agriculture production. Hindawi: Advances in Agriculture, 2021, Article 8924087.
Shukla, P. R., Skea, J., Calvo Buendia, E., Masson-Delmotte, V., Pörtner, H.-O., Roberts, D. C., Zhai, P., Slade, R., Connors, S., van Diemen, R., Ferrat, M., Haughey, E., Luz, S., Neogi, S., Pathak, M., Petzold, J., Portugal Pereira, J., Vyas, P., Huntley, E., Kissick, K., Belkacemi, M., & Malley, J. (Eds.). Climate change and land: An IPCC special report on climate change, desertification, land degradation, sustainable land management, food security, and greenhouse gas fluxes in terrestrial ecosystems. Intergovernmental Panel on Climate Change.
Skendžić, S., Zovko, M., Živković, I. P., Lešić, V., & Lemić, D. (2021). The impact of climate change on agricultural insect pests. Insects, 12(5), 440.
Sparks, T. (2020). Global governance of greenhouse gases.
National Center for Emerging and Zoonotic Infectious Diseases. (2014). Antibiotic resistance from the farm to the table. [Infographic]. Division of Foodborne, Waterborne, and Environmental Diseases.
Tans, P. (2009). An accounting of the observed increase in oceanic and atmospheric CO2 and an outlook for the future. Oceanography, 22(4), 26–35.
Taub, D. (2010). Effects of rising atmospheric concentrations of carbon dioxide on plants. Nature Education Knowledge, 1(8), 21.
U.S. Department of Agriculture. (2024). Soil health principles and practices.
U.S. Environmental Protection Agency. (2017). Climate impacts on agriculture and food supply. Last updated September 23, 2024.
———. (2023). Climate change indicators: Atmospheric concentrations of greenhouse gases. Last updated November 1, 2023.
———. (2024). Overview of greenhouse gases. Last updated November 26, 2024.
U.S. Geological Survey. (2015). EarthWord: Anthropogenic. Last updated September 1, 2015.
Viguera, B., Martínez-Rodríguez, M.R., Donatti, C., Harvey, C. A., & Alpízar, F. (2017). Módulo 2: Impactos del cambio climático en la agricultura de Centroamérica, estrategias de mitigación y adaptación. Proyecto CASCADA. Conservación Internacional and Centro Agronómico Tropical de Investigación y Enseñanza.
Volsi, B., Higashi, G. E., Bordin, I., & Telles, T. S. (2022). The diversification of species in crop rotation increases the profitability of grain production systems. Scientific Reports, 12(1), Article 19849.
Yu, C., Miao, R., & Khanna, M. (2021). Maladaptation of US corn and soybeans to a changing climate. Scientific Reports, 11(1), Article 12351.
Wallinga, D. (2009). Today’s food system: how healthy is it?. Journal of Hunger & Environmental Nutrition, 4(3-4), 251-281.