6 Agriculture, Soil, and Water
Daniel Guarín; Jhony Benavides; and Daniel Ospina
Learning Objectives
At the end of this chapter, students will be able to:
- Identify two fundamental ecosystem services provided by soils.
- Identify the intersection between soils and at least three of the United Nations Sustainable Development Goals.
- Understand the connection between soils and the history of agriculture in developing nations.
- Describe the advantages to soil health of implementing conservation agriculture.
- Identify at least three physical and cultural aspects that make soils a key part of climate change mitigation strategies.
Introduction
Soils are crucial in providing essential ecosystem services such as safe and abundant food, clean water, support for infrastructure, clean air, and biodiversity (Table 1; Keesstra et al., 2016; Minasny et al., 2015). Ecosystem services are defined as the benefits that people obtain from ecosystems and their ecological functions. These include the provision of food and water, regulation of flooding or temperature changes, and even disease control (Mandle et al., 2021).
Soils serve as a medium for plant growth and support all terrestrial life by providing the necessary conditions for their well-being (Lal, 2016). To fully understand the importance of healthy soils, we must view them holistically. This means considering their physical properties, functions, ecosystem services, relationship to other elements of the environment, and social, environmental, economic, political, and cultural dimensions. This interconnectedness must be translated into policies and regulations that promote the sustainable use of soils to foster human well-being and ecosystem health, following the principle of integrality (IDEAM & UDCA, 2015). This chapter explores how soil can play a key role in these interactions and how incorporating soil science into our efforts to achieve the United Nations Sustainable Development Goals (SDGs) is vital.
Table 1. Ecosystem services performed by soils (Weil & Brady, 2017, p. 20).
1. Provisioning (providing goods such as water, food, medicines, lumber, etc.) |
2. Regulating (processes that purify water, decompose wastes, control pests, or modify atmospheric gases) |
3. Supportive (assisting with nutrient cycling, seed dispersal, primary biomass production, etc.) |
4. Cultural (providing spiritual uplift, scenic views, and outdoor recreation opportunities)
5. Foundation (providing a medium for the emplacement of human-designed infrastructure) |
Soil and the United Nations Sustainable Development Goals
Humankind faces many pressing challenges, including poverty reduction, social inclusion, increased equity, education, healthcare, biodiversity conservation, sustainable energy, water security, and climate change adaptation and mitigation (Herrero et al., 2021). These interlinked challenges are embodied in the 2030 Agenda for Sustainable Development adopted by all United Nations (UN) member states in 2015. The agenda is built around 17 Sustainable Development Goals (SDGs; Bruce M et al., 2018; Herrero et al., 2020) that represent a clear challenge to national governments and a wide range of stakeholders (Keesstra et al., 2016).
Many of these goals depend on a better understanding and management of the biophysical systems in which soils play a key role in achieving the desired outcomes (Keesstra et al., 2016; Lal et al., 2021). Six major challenges facing humanity—(i) biodiversity loss, (ii) climate change, (iii) water security, (iv) land degradation, (v) human health, and (vi) food security—are dependent on soils being able to fulfill their natural ecosystem functions. These challenges are interlinked in the UN SDGs (Fig. 1; Keesstra et al., 2016). In this framework, healthy soils are a key element in 11 out of the 17 SDGs (Lal et al., 2021). These include SDG 1 (End Poverty), SDG 2 (Zero Hunger), SDG 3 (Good Health and Well-being), SDG 5 (Gender Equality), SDG 6 (Clean Water and Sanitation), SDG 7 (Affordable and Clean Energy), SDG 9 (Industry Innovation and Infrastructure), SDG 11 (Sustainable Cities and Communities), SDG 12 (Responsible Consumption and Production), SDG 13 (Climate Action), and SDG 15 (Life on Land).
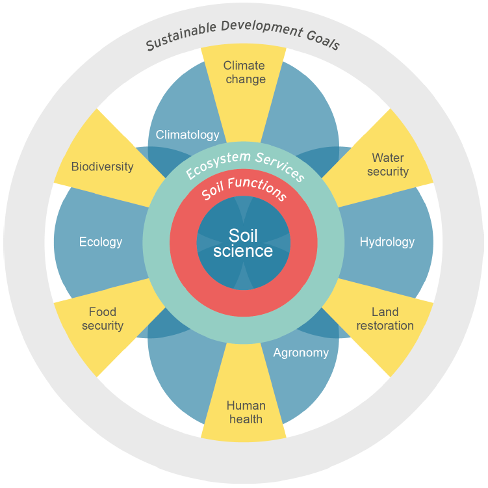
Sustainable Soil and Land Management
Soil degradation caused by land misuse and soil mismanagement is closely connected to global warming, food insecurity, the eutrophication of water (causing millions of fish to die due to lack of oxygen during algal blooms favored by agricultural runoff loaded with chemicals), and the loss of biodiversity (Lal, 2020). Given the impacts of soil degradation on ecosystem health and multiple human activities, it is important for soil scientists to translate their data into a language that policymakers can understand and that civic society and land managers can relate to (Lal, 2020). To address these complex and interconnected issues, we must break down challenges and potential solutions into basic principles of sustainable soil and land management from a soil and ecosystem health perspective.
The basic principles of sustainable soil and land management in agroecosystems include (a) always keeping the soil covered and protected, (b) eliminating or minimizing mechanical perturbation, (c) replacing whatever is harvested or removed (e.g., nutrients, soil organic carbon), (d) enhancing the activity and species diversity of soil biota (e.g., microorganisms, mycorrhizal fungi, and earthworms), (e) integrating crops with livestock and trees into complex farming systems, and (f) recycling all of the byproducts back into the soil (Lal, 2020).
Soil and Climate Change
The negative impacts of climate change on human beings are evident and growing (Van Huynh et al., 2020). One of the key issues discussed by soil scientists is soil’s capacity to sequester carbon, a potential mitigation strategy for anthropogenic global warming (Lal, 2020). The rapid uptake of carbon dioxide removal strategies is mentioned as a key tool to mitigate climate change in the Sixth Assessment Report (AR6) by the International Panel on Climate Change (Riahi et al., 2022).
Soils are exceptional at capturing carbon dioxide (CO2) from the atmosphere. Some carbon dioxide removal (CDR) practices depend on healthy soils. Enhanced weathering, for example, involves the application of powdered rocks such as basalt to agricultural fields. The rocks react with the CO2 in the atmosphere and rainwater to remove the CO2 from the atmosphere and capture it in a mineral form that will not be released for thousands of years (Goll et al., 2021). This practice also improves soil fertility by providing essential plant nutrients to agricultural soils (Goll et al., 2021).
Biochar, produced by the combustion of biomass under low amounts of oxygen, has been hailed as another of the most scalable and effective nature-based solutions to remove atmospheric carbon dioxide (Lehmann et al., 2021). The resulting charred material is characterized by high amounts of very stable carbon that will not be easily degraded by microorganisms and that will remove atmospheric CO2 for tens to hundreds of years (Lehmann et al., 2021). Biochar, when applied to agricultural fields, provides other co-benefits, too: it enhances the soil water holding capacity, stabilizes organic matter, and improves microbial activities (Nair et al., 2017). Given its high porosity and high surface charges, biochar also attracts and retains plant-essential nutrients, prevents plants from absorbing heavy metals and other pollutants (Ramtahal et al., 2019), and relieves soil compaction due to its low bulk density (Nair et al., 2017). Another added benefit of biochar is the reduced need for fertilizer as it holds onto added nutrients, reducing runoff and infiltration of fertilizers that can contaminate downstream water systems (Lehmann et al., 2021).
However, the benefits of using crushed basalt rocks and/or biochar depend on healthy soils in which the amendments will stay in place and benefit from the presence of soil microorganisms and soil nutrients. Predicting the response of soils to climate change and soils’ continued ability to capture carbon is extremely important, given that the top meter of soils globally contains three times as much carbon as is found in the atmosphere (Smith, 2004). Intense soil degradation can lead to this large pool of carbon being released into the atmosphere, increasing the effect of greenhouse gases on global warming. Unfortunately, technical and financial constraints often limit the capacity to sustainably manage soil and water resources in the context of climate change (Roop & St. Martin, 2020).
Natural Landscapes and Agricultural Landscapes
The Co-Benefits Between Conservation and Agriculture
There is growing interest in sustainable agricultural management practices that contribute to food security, counteract soil degradation, and improve climate change resilience (Beuchelt & Badstue, 2013). Conservation agriculture (CA) systems have been adopted by farmers worldwide, mainly those working on large-scale commercial farms (Ngwira et al., 2013). The current adoption rate of CA systems is 0.36% of farms worldwide, with Latin America showing the highest adoption rate at 1.24% (Project Drawdown, 2022). CA systems are based on minimal soil disturbance, crop residue retention, and crop rotation and/or intercrop association with leguminous or cash crops for family use or sale. These rotation crops are increasingly used to replenish and recycle nutrients, increase yield, and reduce production costs (Food and Agriculture Organization, 2011; Ngwira et al., 2013).
Research in Malawi from 2005 to 2011 found that even though CA practices have positive effects on crop productivity, profitability, and soil quality, adoption is still limited by factors outside of the biophysical sphere (Ngwira et al., 2013). Challenges to adoption are still reliant on social dynamics. In the sub-Saharan African context, women farmers adopted CA less and do not adopt it more than men, largely due to gendered barriers including a lack of access to land, machinery, inputs, extension services, and credit facilities (Wekesah et al., 2019). This leads to less exposure to the benefits of CA and a lack of human and technological resources to switch from traditional agriculture to CA (Wekesah et al., 2019).
Increased food production helps boost food availability but does not necessarily foster human development or create enhanced food security (Beuchelt & Badstue, 2013). The social dynamics of smallholder agriculture and the inequity in access to technological, human, and educational tools are still key factors preventing higher CA adoption rates. Thus, future CA research needs to focus on analyzing the potential effects of CA on women and men in smallholder agricultural systems, possible impacts of CA on agricultural yields, and recommendations for future implementation such as the ones described by Brouder and Gomez-Macpherson (2014).
Small-Scale Agricultural Systems: Soil and Smallholder Farmers
Smallholder farmers produce 80% of the developing world’s food on marginal soils constrained by climate-related factors (Roop & St. Martin, 2020). This situation can be exacerbated if soils naturally have a heavy clay texture (>35% clay minerals), resulting in low infiltration rates and inadequate root depth (Roop & St. Martin, 2020).
Roop and St. Martin (2020) describe the soil and water management initiatives, strategic business, and crop management plans of smallholder farmers in Trinidad and Tobago, many of which depend directly on soil health. Healthy soils are fundamental to life on earth (Greiner et al., 2017; Keesstra et al., 2016; Wall et al., 2015), and climate is at the core of many functions important to healthy soils.
Soil quality and soil health are two different concepts. Soil quality is related to soil functions, whereas soil health presents soil as a finite and dynamic living resource and is directly related to plant health (Lal, 2016). Through plant growth, soil health is connected with the health of the animals, humans, and ecosystems within the soil’s domain (Lal, 2016).
Case Study: History of Agriculture in the Valle del Cauca Department, Colombia. Implications and Challenges for Present and Future Food Production in the Region.
Background
Colombia is a diverse country with a significant agricultural sector representing 6.7% of the national GDP (World Bank, 2019). Since the Spanish ruled what was once known as the Viceroyalty of New Granada, the geographical valley of the Cauca River in the Valle del Cauca department has been recognized as an outstanding region for agricultural production due to the following characteristics:
- flat topography (more than 200,000 hectares between the central and western ranges of the Andes);
- deep and fertile soils (Mollisols and Vertisols mainly); and
- warm and stable climate parameters all year round (23°C average, never above 35°C, never under 15°C, precipitation in the range of 1000 mm, day/night almost 12h/12h).
The Valle del Cauca department (Fig. 2) has an area of 22,140 square kilometers (2,214,000 ha), corresponding to 1.95 % of the Colombian territory. It consists of 42 municipalities: 21 on the left bank of the Cauca River, 20 on the right, and the Buenaventura municipality on the Pacific coast.
The department is characterized by intensive agriculture, with the main crop of sugar cane covering approximately 200,000 ha (around 50% of the arable land in the area) for the production of sugar and bioethanol (Morales et al., 2011; Villamizar & Brown, 2016). The Cauca River is located between the west and central Andean ranges in Colombia and is one of the country’s two main rivers. It flows from its source in the Colombian Massif for approximately 1,350 km, draining a watershed of 63,300 km2 to its confluence with the Magdalena River and finally flowing into the Caribbean Sea (Villamizar & Brown, 2016).
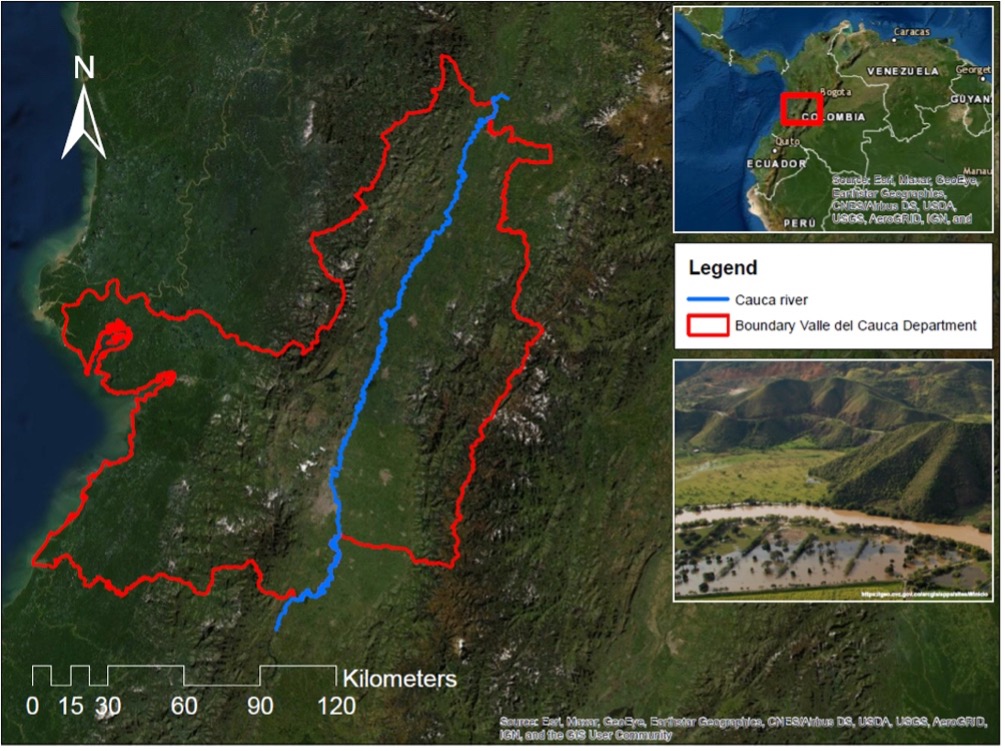
In a survey of 37 contrasting watersheds throughout the Valle del Cauca department, the Agustín Codazzi Colombian Geographic Institute and Valle del Cauca’s Regional Autonomous Corporation found that there are five thermal floors and five humidity provinces. These result in 14 distinct climate regimes , with the humid temperate climate (39.66%) dominating, followed by the dry temperate climate (13.89%), humid cold (10.94%), and very humid cold (8.10%) (Fig. 3).
These institutions also report that the soils are mostly deep (46.8%) and moderately deep (20.3%). The major limitations to crop growth are the effective zoot zone depth, high rock fragment content (52.0%), and impermeable clay and/or argillic horizons (21.6%). The soils are characterized as well drained (92.8%), with dominant moderately fine (39.2%) and fine (38.5%) textures, resulting in a natural fertility score ranging from moderate (34.1%) to high (32.1%) (Instituto Agustín Codazzi & Corporación Autónoma Regional del Valle del Cauca, 2014).
A ![]() |
B ![]() |
C ![]() |
Fig. 3. Contrasting landscapes in the Valle del Cauca region: (a) coffee, plantain, and medicinal herbs crops at the western mountain range, (b) Buenaventura Port, and (c) mountain landscape at the Palmira Municipality (Instituto Agustín Codazzi & Corporación Autónoma Regional del Valle del Cauca, 2014).
Without significant mining activities, early Spanish settlers established big farms known as haciendas. These farms were initially worked by indigenous peoples and later by African slaves. However, due to poorly developed commercial networks and infrastructure at the time, the commodities were mainly meant to satisfy the demand of the cities nearby (Popayán, Cali, Buga, Cartago). This led to a self-sufficient agricultural system.
After Colombia gained its independence from the Spanish Empire in 1819, the liberalization of the economy and increased demand led to several peaks in tobacco cultivation, which in turn generated important tax revenues (Cortes-Ortiz, 2010). However, over time, the country’s tobacco production fell behind that of other countries (like Cuba and the United States), leaving increased acreage for sugar cane. Since then, sugar cane cultivation has dominated the upper Cauca River Valley (Fig. 4).
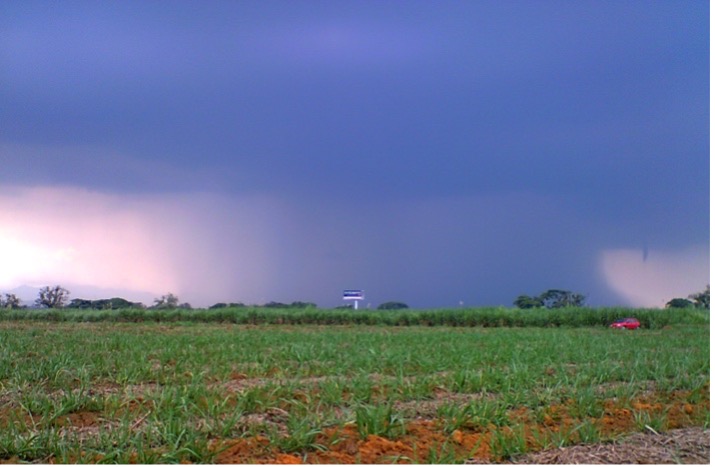
Since the beginning of the nineteenth century, the population of Valle del Cauca has grown significantly. The hillsides and plateaus of the central and western ranges of the Andes suffered a steady process of colonization by jobless and landless people from the haciendas. This led to the creation of multiple new towns and the occupation of the surrounding land in what is known as Antioquia’s colonization. Despite the challenges these lands pose for agriculture, such as steep slopes and thinner soils than those in the Cauca River Valley, the new settlers successfully implemented family-owned and operated agricultural systems based on coffee and plantain crop production.
By the middle of the twentieth century, the production of maize and cotton had become important for the plains, but the imports from North America eventually devastated this revenue stream. These historical processes have shaped the present-day agriculture in the Valle del Cauca department: today there are large agro-industrial sugar cane plantations in the flood plains on both sides of the Cauca River and small subsistence and/or market-oriented coffee farms in the hillsides of the central and western ranges of Andes.
Due to intense farming over the years on the fertile and productive soils of the river valley, soil degradation processes such as salinization, desertification, and increased use of agrichemicals , have occurred (Cortes-Ortiz, 2010) . This soil degradation poses a risk to healthy soils’ ability to provide vital ecosystem services such as growing food, regulating flooding, capturing atmospheric carbon, and filtering contaminants that end up in the Cauca River, promoting contamination further downstream.
Enhanced soil management practices such as crop rotations, the absence or reduction of tillage, and cover crops when farms are left fallow, all of which are practices that fall under the CA umbrella, could help mitigate soil the degradation seen in the Cauca River Valley.
Modern-Day Agriculture in the Valle del Cauca Department
Located in the southwest corner of Colombia and only 3° to 4° degrees north of the equator, the department receives intense and almost invariable sunlight throughout the year. These conditions are very advantageous for sugar cane cultivation. More than 90% of the sugar cane grown in Colombia is produced in this region, where it reaches yields of about 120 tons per hectare. Almost 10% of the cane weight is sucrose, making the final yield around 12 tons of sugar per hectare. This is the highest yield in the world, comparable only to Australia’s.
In the past 15 years, the sugar mill companies (11 in total, covering more than 200,000 hectares) have successfully attempted to diversify their portfolios through the production of bioethanol. Several sugar mills also generate considerable amounts of electricity by burning the biomass from the ground cane residue, making them co-generators of electricity to the national system. The cellulose of this biomass is also used for paper fabrication.
Virtually all the highly productive flat areas alongside the Cauca River are occupied by sugar cane fields. The remaining economically important crops have been displaced to the hillsides of the Andes Mountains (Fig. 5). The mountain range’s climates allow for the adaptation of various crops. The main crop is coffee, followed by bananas, plantains, sour-lime/orange/tangerines, avocados, guavas, grapevines, pineapple, orchards, and aromatic and medicinal plants, among others (Fig. 6).
Coffee growing is still seen as a livelihood for farmers. However, due to severe fluctuations in international prices, the trend is to switch to more market-steady crops, like avocados, citrus fruits, or pineapple, or even greenhouse crops like tomatoes.
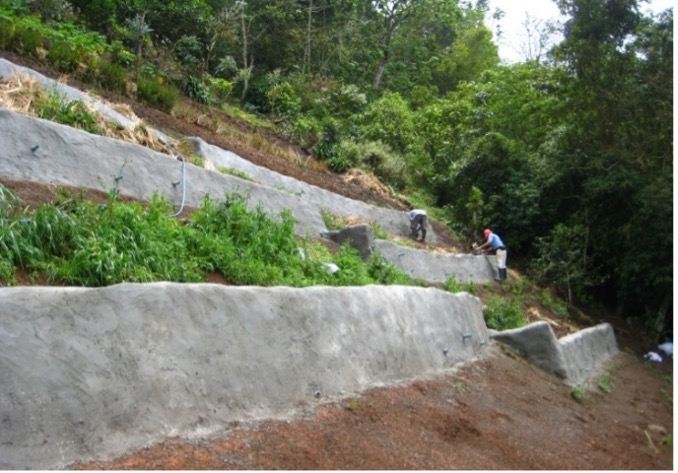
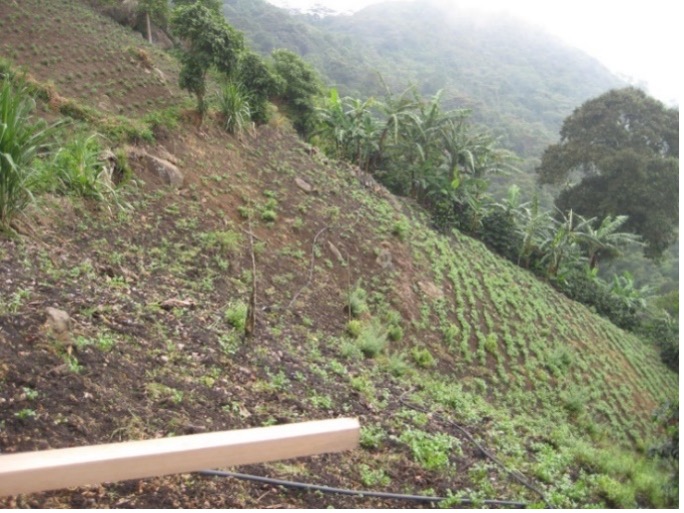
Agriculture in the Pacific coastal lowlands of Valle del Cauca is marginal and consists mostly of subsistence crops like plantains and cassava, although cacao and chontaduro (a local palm whose fruit is appreciated in the cities as a traditional snack) are produced in a few hundred hectares. The limitation in this sub-region is its heavy precipitation , which reaches more than 6,000 mm per year, causing poor fertility of the soils . Excessive rainfall tends to remove essential nutrients such as calcium, potassium, and magnesium from the soil, leaving behind a soil profile with a low concentration of nutrients necessary for plant growth (Weil & Brady, 2017). Additionally, most of the area is virgin rainforest and owned collectively by Afro-descendants and indigenous communities not interested in big agri-business ventures.
Challenges in Soil Management
One of the most relevant challenges in soil management in Valle del Cauca and Colombia is soil erosion caused by water runoff in poorly managed fields of the Andes Mountains. This deterioration is worsened by cattle ranching as a means of weed control. This leads to poorly covered soils that barely retain topsoil and results in soil compaction (Morales et al., 2011). These issues have been well described and analyzed (Guzman et al., 2019; IDEAM & UDCA, 2015), but the lack of enforcement and no access to land for small farmers force small farmers to settle in the steepest parts of the mountains. Meanwhile, the fact that cattle ranching is more profitable than letting the natural vegetation recover makes it difficult to tackle these social and environmental issues.
Another problem is the lack of an updated spatial and temporal classification of land use and land cover maps, which limits the adoption of enhanced soil and land management practices (Valencia-Payan et al., 2018). In Colombia and other developing countries, most of the territory is covered by general soil surveys at a 1:100,000 scale. However, there is increasing demand for up-to-date, detailed information that is costly and time-consuming to obtain (Zinck et al., 2015). Soil salinization and sodification, which affect mostly sugar cane and cattle lands and are associated with soil compaction, are other soil-related issues in Valle del Cauca. A summary of soil degradation components is presented in Figure 7.
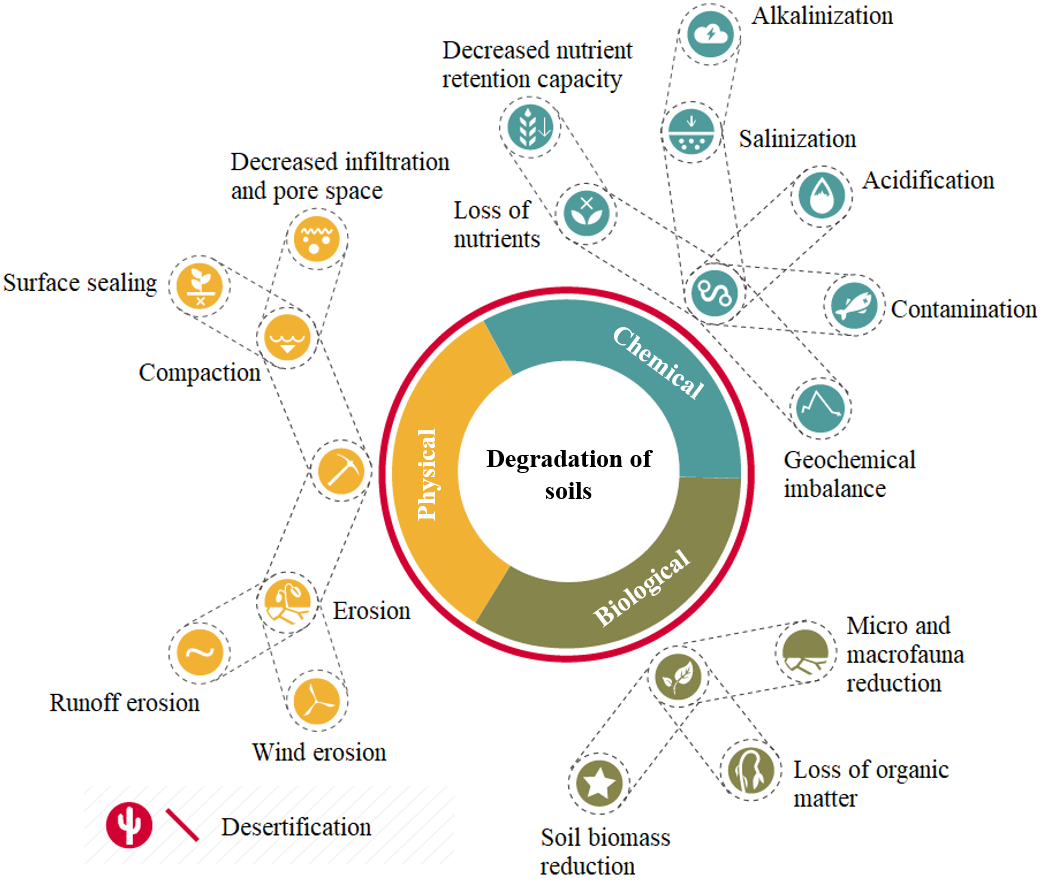
Perspectives and Challenges for Researchers and Extension Specialists
Agri-business-oriented and commodity crops such as sugar cane and coffee have lobby support from institutions like Asocaña (sugar cane sector association) and Fedecafé (national federation of coffee farmers). These organizations have strong ties at the highest levels of government and look after the interests of their members. They have achieved important economic agreements throughout the years and support scientific institutions such as Cenicaña (sugar cane research center) and Cenicafé (coffee research center), which have released crop varieties with enhanced yield and adaptation to the soils and climate of the region. Other research lines that have contributed to improving cultivation include plant nutrition strategies, irrigation, drainage, water balance at the crop level, plant ecophysiology, pest management, and post-harvest techniques.
However, other crops have received little research support or lobby ing at the highest levels of government. Research support is limited to university programs/projects and the public agri-research institution Agrosavia. The budget is restricted for all the other crops with less economic relevance, making it unlikely there will be a true diversification of crops to divert attention from traditional sugar cane. Moreover, financial incentives to adopt CA practices, such as crop rotations, reduced tillage, and fallow, fail to attract agro-industrial growers who prefer a constant revenue stream, ignoring the potential adverse effects of such intensive practices on future soil health.
Extension activities, once in charge of the so-called units of agricultural assistance (UMATAS), are scarce. Unlike U.S. universities, Colombian universities have not been committed to any extension activities, which is partly why many farmers see universities as occupying a different realm and making no visible contribution to their progress. As a result, most farmers rely on their knowledge or that of their neighbors and continue using conventional practices.
The challenge for researchers and extension specialists lies in providing technical support and access to funding to increase the adoption rates of CA in developing countries. Soil degradation is a silent and slow threat that is hard for non-specialists to observe and thus often ignored. This poses a threat not only to farmers’ revenue streams but also to everyone who depends on healthy soils and the ecosystem services they provide. Shedding light on the need for healthy soils to accomplish the UN SDGs should be a top priority for researchers and agricultural extensionists.
Conclusion
Soils provide a wide array of ecosystem services crucial for agriculture and human interaction with our environment. They provide a growing medium for food, clean and sufficient water, a foundation for infrastructure, and a buffer against greenhouse gases and climate change. However, rapid soil degradation threatens the future of agriculture and access to basic resources. This is especially true in developing regions where access to the technical, financial, and academic resources essential for sustainable agriculture and land-use practices is lacking.
The uneven distribution of resources between the developed and developing worlds affects not only soil but also multiple other dimensions of human life. It exposes vulnerable communities and ecosystems to future climate change, soil degradation, and unproductive agricultural systems. The UN SDGs consider every aspect of human development, addressing global agricultural issues such as food security, food safety, and ecosystem health. To accomplish these goals, research and advocacy efforts must aim toward enhanced management practices that promote healthy soils. Soil health should be among the top priorities for future agricultural, economic, industrial, and environmental policy.
To holistically address these present and future challenges, it is crucial to understand healthy soils both as a shared space in which we interact with our biophysical sphere and as a medium of human sustenance. After all, soil health means human health.
Further Exploration
- United Nations Sustainable Development Goals
- Agroecosystems and Sustainable Landscapes (International Centre of Tropical Agriculture)
- Soils Portal (Food and Agriculture Organization of the United Nations)
- Living Soil Film (Soil Health Institute): An exploration of how soil health means human health
Check Your Knowledge!
- Name at least two ecosystem services that are connected to soils.
- Name some of the SDGs that are connected to soil health.
- How is smallholder agriculture connected to soil conservation?
- Name at least three ways in which soils are a key tool for mitigating climate change.
References
Beuchelt, T. D., & Badstue, L. (2013). Gender, nutrition- and climate-smart food production: Opportunities and trade-offs. Food Security, 5(5), 709–721. https://doi.org/10.1007/s12571-013-0290-8
Brouder, S. M., & Gomez-Macpherson, H. (2014). The impact of conservation agriculture on smallholder agricultural yields: A scoping review of the evidence. Agriculture, Ecosystems and Environment, 187, 11–32. https://doi.org/10.1016/j.agee.2013.08.010
Campbell, B. M., Hansen, J., Rioux, J., Stirling, C. M., Twomlow, S., & Wollenberg, E. (L.). (2018). Urgent action to combat climate change and its impacts (SDG 13): Transforming agriculture and food systems. Current Opinion in Environmental Sustainability, 34, 13–20. https://doi.org/10.1016/j.cosust.2018.06.005
Cortes-Ortiz, B. (2010). Descripción Socioambiental Del Suelo En El Valle Geográfico Del Río Cauca: El Caso De La Agroindustria Azucarera. Luna Azul, 31, 41–57. https://doi.org/10.17151/luaz.2010.31.5
Food and Agriculture Organization. (2011). Conservation agriculture. United Nations.
Goll, D. S., Ciais, P., Amann, T., Buermann, W., Chang, J., Eker, S., Hartmann, J., Janssens, I., Li, W., Obersteiner, M., Penuelas, J., Tanaka, K., & Vicca, S. (2021). Potential CO2 removal from enhanced weathering by ecosystem responses to powdered rock. Nature Geoscience, 14(8), 545–549. https://doi.org/10.1038/s41561-021-00798-x
Greiner, L., Keller, A., Grêt-Regamey, A., & Papritz, A. (2017). Soil function assessment: Review of methods for quantifying the contributions of soils to ecosystem services. Land Use Policy, 69, 224–237.
Guzman, C. D., Hoyos-Villada, F., Da Silva, M., Zimale, F. A., Chirinda, N., Botero, C., Morales Vargas, A., Rivera, B., Moreno, P., & Steenhuis, T. S. (2019). Variability of soil surface characteristics in a mountainous watershed in Valle del Cauca, Colombia: Implications for runoff, erosion, and conservation. Journal of Hydrology, 576(June), 273–286. https://doi.org/10.1016/j.jhydrol.2019.06.002
Herrero, M., Thornton, P. K., Mason-D’Croz, D., Palmer, J., Benton, T. G., Bodirsky, B. L., Bogard, J. R., Hall, A., Lee, B., Nyborg, K., Pradhan, P., Bonnett, G. D., Bryan, B. A., Campbell, B. M., Christensen, S., Clark, M., Cook, M. T., de Boer, I. J. M., Downs, C., … West, P. C. (2020). Innovation can accelerate the transition towards a sustainable food system. Nature Food, 1(5), 266–272. https://doi.org/10.1038/s43016-020-0074-1
Herrero, M., Thornton, P. K., Mason-D’Croz, D., Palmer, J., Bodirsky, B. L., Pradhan, P., Barrett, C. B., Benton, T. G., Hall, A., Pikaar, I., Bogard, J. R., Bonnett, G. D., Bryan, B. A., Campbell, B. M., Christensen, S., Clark, M., Fanzo, J., Godde, C. M., Jarvis, A., … Rockström, J. (2021). Articulating the effect of food systems innovation on the Sustainable Development Goals. The Lancet: Planetary Health, 5(1), e50–e62. https://doi.org/10.1016/S2542-5196(20)30277-1
IDEAM & UDCA. (2015). Estudio nacional de la degradación de suelos por erosión en Colombia. Please write out acronyms at beginning of citation and add publication info
Instituto Agustín Codazzi, & Corporación Autónoma Regional del Valle del Cauca. (2014). Levantamiento Semidetallado de Suelos Escala 1:25,000 de las cuencas priorizadas por la Corporación Autónoma Regional del Valle del Cauca – CVC.
Keesstra, S. D., Bouma, J., Wallinga, J., Tittonell, P., Smith, P., Cerdà, A., Montanarella, L., Quinton, J. N., Pachepsky, Y., & Van Der Putten, W. H. (2016). The significance of soils and soil science towards realization of the United Nations Sustainable Development Goals. Soil, 2(2), 111–128. https://soil.copernicus.org/articles/2/111/2016/
Lal, R. (2016). Soil health and carbon management. Food and Energy Security, 5(4), 212–222.
Lal, R. (2020). Managing soils for resolving the conflict between agriculture and nature: The hard talk. European Journal of Soil Science, 71(1), 1–9. https://doi.org/10.1111/ejss.12857
Lal, R., Bouma, J., Brevik, E., Dawson, L., Field, D. J., Glaser, B., Hatano, R., Hartemink, A. E., Kosaki, T., Lascelles, B., Monger, C., Muggler, C., Ndzana, G. M., Norra, S., Pan, X., Paradelo, R., Reyes-Sánchez, L. B., Sandén, T., Singh, B. R., … Zhang, J. (2021). Soils and sustainable development goals of the United Nations: An International Union of Soil Sciences perspective. Geoderma Regional, 25, Article e00398. https://doi.org/10.1016/j.geodrs.2021.e00398
Lehmann, J., Cowie, A., Masiello, C. A., Kammann, C., Woolf, D., Amonette, J. E., Cayuela, M. L., Camps-Arbestain, M., & Whitman, T. (2021). Biochar in climate change mitigation. Nature Geoscience, 14(12), 883–892. https://doi.org/10.1038/s41561-021-00852-8
Mandle, L., Shields-Estrada, A., Chaplin-Kramer, R., Mitchell, M. G. E., Bremer, L. L., Gourevitch, J. D., Hawthorne, P., Johnson, J. A., Robinson, B. E., Smith, J. R., Sonter, L. J., Verutes, G. M., Vogl, A. L., Daily, G. C., & Ricketts, T. H. (2021). Increasing decision relevance of ecosystem service science. Nature Sustainability, 4(2), 161–169. https://doi.org/10.1038/s41893-020-00625-y
Minasny, B., Finke, P., Stockmann, U., Vanwalleghem, T., & McBratney, A. B. (2015). Resolving the integral connection between pedogenesis and landscape evolution. Earth-Science Reviews, 150, 102–120. https://doi.org/10.1016/j.earscirev.2015.07.004
Morales, E. M., Ramirez, J. A., Albán, Á., Escobar, B. Y., García, L. F., & Peña, M. E. (2011). Compactación de suelos cultivados con caña de azúcar en la zona sur del Valle del Cauca. Parte I. Acta Agronómica, 60, 245–252.
Nair, V. D., Nair, P. K. R., Dari, B., Freitas, A. M., Chatterjee, N., & Pinheiro, F. M. (2017). Biochar in the agroecosystem–climate-change–sustainability nexus. Frontiers in Plant Science, 8. https://doi.org/10.3389/fpls.2017.02051
Ngwira, A. R., Thierfelder, C., & Lambert, D. M. (2013). Conservation agriculture systems for Malawian smallholder farmers: Long-term effects on crop productivity, profitability and soil quality. Renewable Agriculture and Food Systems, 28(4), 350–363. https://doi.org/10.1017/S1742170512000257
Project Drawdown. (2022). Conservation agriculture. Drawdown Solutions Library. https://www.drawdown.org/solutions/conservation-agriculture
Ramtahal, G., Umaharan, P., Hanuman, A., Davis, C., & Ali, L. (2019). The effectiveness of soil amendments, biochar and lime, in mitigating cadmium bioaccumulation in Theobroma cacao L. Science of The Total Environment, 693, Article 133563. https://doi.org/10.1016/J.SCITOTENV.2019.07.369
Riahi, K., R. Schaeffer, J., Arango, K., Calvin, C., Guivarch, T., Hasegawa, K., Jiang, E., Kriegler, R., Matthews, G. P., Peters, A., Rao, S., Robertson, A. M., Sebbit, J., Steinberger, M., & Tavoni, D. P. van V. (2022). Mitigation pathways compatible with long-term goals. In P. R. Shukla, J. Skea, R. Slade, A. Al Khourdajie, R. van Diemen, D. McCollum, M. Pathak, S. Some, P. Vyas, R. Fradera, M. Belkacemi, A. Hasija, G. Lisboa, S. Luz, & J. Malley (Eds.), Climate Change 2022: Mitigation of Climate Change. Contribution of Working Group III to the Sixth Assessment Report of the Intergovernmental Panel on Climate Change. Cambridge University Press, Cambridge, UK. https://doi.org/10.18356/9789210012973c007 Content at link doesn’t match citation – double-check?
Roop, R., & St. Martin, C. C. G. (2020). Building climate resilience of smallholder family farms by implementing integrated soil and water management strategies in Trinidad and Tobago. In W. L. Filho, J. M. Luetz, & D. Ayal (Eds.), Handbook of Climate Change Management (pp. 1–29). Springer. https://doi.org/10.1007/978-3-030-22759-3_92-1
Smith, P. (2004). Soils as carbon sinks: The global context. Soil Use and Management, 20(2), 212–218. https://doi.org/10.1111/j.1475-2743.2004.tb00361.x
Valencia-Payan, C., Pencue-Fierro, E. L., Figueroa-Casas, A., & Corrales, J. C. (2018). Multitemporal land cover change analysis in the Upper Cauca Basin in Colombia. Advances in Intelligent Systems and Computing, 687, 209–222. https://doi.org/10.1007/978-3-319-70187-5_16
Van Huynh, C., Pham, T. G., Nguyen, T. Q., Nguyen, L. H. K., Tran, P. T., Le, Q. N. P., & Nguyen, M. T. H. (2020). Understanding indigenous farming systems in response to climate change: An investigation into soil erosion in the mountainous regions of Central Vietnam. Applied Sciences (Switzerland), 10(15). https://doi.org/10.3390/app10155091
Villamizar, M. L., & Brown, C. D. (2016). Modelling triazines in the valley of the River Cauca, Colombia, using the annualized agricultural non-point source pollution model. Agricultural Water Management, 177, 24–36. https://doi.org/10.1016/j.agwat.2016.06.010
Wall, D. H., Nielsen, U. N., & Six, J. (2015). Soil biodiversity and human health. Nature, 528(7580), 69.
Weil, R. R., & Brady, N. C. (2017). The nature and properties of soils (15th ed.). Pearson Education Limited.
Wekesah, F. M., Mutua, E. N., & Izugbara, C. O. (2019). Gender and conservation agriculture in sub-Saharan Africa: A systematic review. International Journal of Agricultural Sustainability, 17(1), 78–91. https://doi.org/10.1080/14735903.2019.1567245
World Bank. (2019). Agriculture, forestry, and fishing, value added (% of GDP) [Graph].
Zinck, J. A., Metternicht, G., Bocco, G., & Del Valle, H. F. (Eds.) (2015). Geopedology: An integration of geomorphology and pedology for soil and landscape studies. Springer. https://doi.org/10.1007/978-3-319-19159-1