7 Agroecology and Biodiversity Conservation
Nina Camillone and Jacob Johnson
Learning Objectives
At the end of the chapter, students will be able to:
- Explain the importance of biodiversity in agricultural systems by giving examples of ecosystem services.
- Describe interactions between agricultural practices and biodiversity.
- Provide region-specific examples of biodiversity conservation in agroecological systems.
Introduction to Basic Concepts
Biodiversity
It might seem that all a seed needs to grow is fresh air, sunlight, fertile soil, and water—but a closer look reveals that there is a team of other organisms that support and protect the plant as it grows. Consider a hypothetical maize (Zea mays) crop. The essential nitrogen-containing nutrients it absorbs from the soil were deposited there by the nitrogen-fixing bacteria (Bradyrhizobium spp.) that lived in the leguminous soybean roots (Glycine max) that grew in the same field the previous season. The soil is rich in nutrients because it was fertilized with manure from cows (Bos taurus) and other livestock species. The maize plant’s ability to mine phosphorus and other nutrients from the soil is enhanced by the symbiosis between its roots and an arbuscular mycorrhizal fungus (Glomus fasciculatum). Molasses grass (Melinis minutiflora) intercropped with the maize both repels destructive stem borers (including Sesamia calamistis) and harbors a natural parasite of this pest (Cotesia sesamiae). This network of agriculture-associated organisms, together with the nonliving environment, is part of an agroecosystem. The study of agroecosystems in their environmental and socioeconomic contexts is known as agroecology.
Agroecosystem: Human-managed ecosystem with the purpose of food and fiber production.
Agroecology: The study of agroecosystems in their environmental and socioeconomic contexts.
Around the world, traditional agricultural systems have been developed to harness and manage biodiversity for food production. Today, agricultural intensification continues to alter interspecies relationships in unpredictable ways that disrupt the provisioning of critical ecosystem services. This chapter discusses biodiversity conservation and management of ecosystem services within agricultural systems. Case studies from Zambia, Malawi, Zimbabwe, and South Africa illustrate the multiple levels of biodiversity within agroecosystems, including the genetic level (crop breeding), the species level (crop selection), the ecosystem level (agroecosystem management), and the ecosystem boundary level (forestland conservation). At all levels of biodiversity, human values and behavior interact with ecosystems and the services they provide.
Introduction to an Agroecologist
Dr. Tafadzwanashe Mabhaudhi
Biodiversity
Earth is home to an amazing variety of life. Biological diversity, or biodiversity, is the term most commonly used to describe this variety. Living organisms, including plants, animals, and microbes, populate almost every environment on the planet. They interact and form complex associations in terrestrial environments like forests, grasslands, deserts, cities, and farmlands, as well as aquatic environments such as rivers, wetlands, estuaries, and oceans (Leemans & De Groot, 2003).
Biodiversity is complex. Scientists who study biodiversity seek to understand this complexity at multiple levels. Diversity ranges from the microscopic level, such as the genetics of individual organisms, to the landscape level, such as communities of different organisms (Gaston & Spicer, 2013). Scientists classify each unique organism into a species according to its genetic makeup, behavior, and ancestral history.
Biodiversity: The variety of living organisms.
Levels of biodiversity:
- Genetic Diversity: The genes and chromosomes that make up individuals, as well as the genetic variation between individuals and between populations
- Organismal Diversity: Organisms, their relatives, and their ancestral history.
- Ecological Diversity: Populations of organisms and the different habitats that support them.
All species require energy, water, and nutrients to maintain life and reproduce. Each species accesses energy, water, and nutrients through unique means. Generally, species of terrestrial plants derive energy from the sun, and water and nutrients from the soil. Animals obtain energy and nutrients from consuming other organisms (“food”) and water from free-standing water bodies. Microorganisms, including bacteria, algae, protozoa, fungi, and viruses, are resourceful in obtaining nutrients through soil, water, other organisms, and the decomposition of organic materials (Gaston & Spicer, 2013; Leemans & De Groot, 2003).
Ecosystems and Ecosystem Services
In the physical environment, organisms interact to produce, transfer, and recycle energy, water, and nutrients. Organisms may interact through competition, herbivory, predation, parasitism, or mutualistic relationships. This network of organisms, the physical environment, and their interactions is known as an ecosystem. Within ecosystems, the processes that regulate the availability of energy, water, and nutrients are known as ecosystem functions (Leemans & De Groot, 2003).
Ecosystem: living plant, animal, and microorganism communities and their non-living environment interacting as a functional unit.
Ecosystem Services: the benefits that humans obtain from ecosystems.
Types of ecosystem services:
- Provisioning Services: material products obtained from ecosystems. Examples include food, water, and fuelwood. Explore the FAO website to learn more.
- Regulating Services: benefits obtained from ecosystem processes (e.g., processes that maintain the quality of air and soil or control floods and disease). Explore the FAO website to learn more.
- Supporting Services: services necessary for the maintenance of all other ecosystem services. Examples include soil formation and nutrient cycling. Explore the FAO website to learn more.
- Cultural Services: non-material benefits that humans obtain from ecosystems. These include religious and spiritual benefits, recreation, ecotourism, education, and cultural heritage. Explore the FAO website.
Change in biodiversity influences ecosystem function. The degree of influence varies depending on the species and function under observation. Scientists have only recently begun understanding the linkages between species loss and ecosystem functions. In some cases, many species drive an ecosystem’s function. Therefore, a loss of one species may not necessarily lead to a decline in ecosystem function. In other cases, only one or two species are responsible for an ecosystem function. In this case, if the species is lost, the essential ecosystem function may be lost. The possibility of significant losses of function increases as more species are lost and redundancy is reduced (Leemans & De Groot, 2003).
Many ecosystem functions are essential for the survival of human beings. “Wait! Human beings?” you may ask. Yes! Humans make up an important part of ecosystems. Like all species, humans depend on ecosystems for water, energy, and food to survive. The ecosystem functions directly linked to human wellbeing are known as ecosystem services. Ecosystem services are wide-ranging. We encourage you to explore the Food and Agriculture Association’s website to learn more about ecosystem services and examples of services that are key to international agricultural development. Links are provided in the text box.
The examples available on the Food and Agriculture Association’s website all support this key point: human activity influences the health of ecosystems and the flow of ecosystem services. Human activity may enhance a particular service while simultaneously degrading another service. As ecosystem managers, humans are often chiefly concerned with the stability of a particular service and the resilience of ecosystems in the face of external shocks or disturbances (Galluzzi et al., 2011).
Let’s examine some principles and specific examples of how biodiversity in human-managed agroecosystems provisions critical ecosystem services that support plant, animal, and human health.
Biodiversity Within Agroecosystems
From an agroecological perspective, agriculture is an organized way in which humans manage natural processes and harness ecosystem services for producing food, fiber, fuel, medicines, and other goods for human consumption. The challenge of a well-managed agroecosystem is to harvest these goods according to human needs without compromising ecological functions. Agroecology, defined earlier in this chapter as the study of agroecosystems in their environmental and socioeconomic contexts, is an essential tool in optimizing this balance between extracting goods and preserving ecosystem services. As a science, agroecology applies ecological theory to the management of agricultural systems (Pretty et al., 2011). It emphasizes the interrelatedness of all agroecosystem components and the complex dynamics of ecological processes (Alteri, 2002).
Since modern agricultural practices often unsustainably exploit ecosystem services, there is a need for farmers, researchers, policy planners, and conservationists to reconsider the relationships between agricultural production and biodiversity conservation. Sustainable land management strategies must take into account how to balance economic pressures to maximize agricultural productivity with the reality that habitat loss and the exploitation of natural ecosystems are destructive to the earth’s environment, agricultural productivity, and human wellbeing (Galluzzi et al., 2011). For example, coffee producers may clear the land of trees to intensify and expand coffee production. However, this deforestation destroys habitats for pollinators and natural predators of coffee berry borers such as birds, often leading to the increased use of toxic chemical pesticides and in turn promoting pesticide resistance (Chain-Guadarrama et al., 2019). Thus, the long-term optimization of agricultural productivity requires consideration of the impacts of agricultural practices on the biodiversity of crop and non-crop species within agroecosystems.
The rest of this section considers several levels of biodiversity management and conservation within agroecosystems: genetic diversity within species, the integration of diverse agricultural species, and the significance of non-crop species in agroecosystems both above and below ground.
Genetic Diversity: A single species can include many breeds or varieties.
Traditionally, all varieties of agricultural species were landraces, local crops, or animal breeds adapted explicitly to a particular location. A crop that a group of people has cultivated for generations, bred using traditional methods, and incorporated into their cultural heritage is typically a landrace. Its characteristics are uniquely suited to its environment of origin. See Zeven (1998) for further discussion of landraces.
In the modern period of agricultural development, also known as the Green Revolution, more farmers have begun buying and growing newly developed and improved seeds representing a few crop species rather than the many traditional crops and varieties that they planted before. An improved breed is one that has been subjected to modern breeding programs to combine desirable qualities from various landraces. Similarly, a hybrid is a strategic cross between two inbred varieties made to exhibit superior traits. A key figure in advancing improved seed varieties is Norman Borlaug (1914–2009), who has been called the father of the Green Revolution. He won the Nobel Peace Prize in 1970 for his pioneering work in Mexico developing improved wheat varieties. The applications of such varieties have dramatically increased crop yields and improved food security status for populations around the world.
However, improved varieties are not readily available to everyone, especially in the Global South, due to a lack of applicable research, market access, knowledge, and/or monetary capital. Additionally, improved seeds are typically selected for highly fertile environments that use fertilizers, herbicides, and pesticides; they are not adapted to a wide range of environments. Thus, they may not be productive without costly agrochemical inputs that may be inaccessible or available only in limited quantities or at particular times in many locations around the world. Improved varieties must be specifically developed for the local environment to match the hardiest landraces in adverse conditions. Meanwhile, a drawback of hybrids is that they are not open-air-pollinated as landraces are, so farmers cannot own their seed lines. While all hybrids of the same type are uniform, many of their offspring revert to the less desirable characteristics of the original parent varieties, forcing a farmer to buy new hybrid seeds each year for the best results.
Excessive replacement of landraces with improved seeds or hybrids threatens to destroy cultural heritage and the genetic diversity that makes landraces well-suited to their environment. This phenomenon is called genetic erosion. For these reasons, landraces may be a better option for a farmer or farming community depending on environmental and financial circumstances. For a discussion of how improved seeds and chemical fertilizers are deployed in African agriculture, see Ariga et al. (2019). For a discussion of the preservation of landraces, see Dwivedi et al. (2016). The science and application of genetically modified organisms is covered in Chapter 10. See the vignette for a discussion of traditional cropping systems that favor indigenous crops and landraces over hybrids and their role in food security and climate change adaptation.
Indigenous Crops
Dr. Tafadzwanashe Mabhaudhi
A lot of my research is focused on understanding African indigenous crops and the potential of these crops to improve productivity in marginal agroecosystems. Marginal agroecosystems in semi-arid areas have inherently low productivity due to low soil fertility and low water availability. These agroecosystems are primarily cultivated by farmers out of necessity for subsistence. We are interested in indigenous crops that are not heavy nutrient miners but instead replenish soil nutrients. Examples are bambara nut, cow peas, and pigeon peas. Over time, these crops strengthen soil structure, which in turn improves the water retention capacity of the soil. As legumes, they also fix nitrogen, reducing the load on mining soil nutrients and decreasing dependence on external fertilizer inputs. In terms of water, indigenous crops are native to the semi-arid environment and have adapted to the low-water conditions. This means they do not require supplementary irrigation and their risk of failing is much lower than that of other crops. Thus, indigenous crops can introduce a new level of stability for farmers cultivating marginal ecosystems.
Crop Diversity: Species with complementary functions can support each other.
Conventional modern agricultural systems that have been developed during the Green Revolution typically intensify the production of a single crop using a package of practices, including improved seed, inorganic fertilizers, pesticides, and irrigation to create “ideal” conditions. This practice of cultivating a single crop in a field is termed monocropping, and the same crop grown in a field over successive years is described as a monoculture. However, in developing regions of the world, all the aspects of this package are often not readily available or financially accessible, rendering any implementation much less effective than it might otherwise be. Such a system may also be much less stable in the face of disturbances such as novel or resistant pests, droughts, and floods.
Many traditional cropping systems around the world incorporate more than one species per field in a complementary growth strategy termed intercropping or polyculture. Studies have shown higher total production per land unit when multiple crops are integrated rather than grown separately (Daryanto et al., 2020). Intentional mixtures can deter the growth of unwanted weed species and in some cases, the spread of pests and diseases (Malézieux et al., 2009). In agroforestry systems, tree species provide shade and/or other benefits for crops growing in the understory. The trees may or may not yield their own products, such as fruit or timber.
Crop diversity within an agroecosystem is significant not only spatially but also temporally. A primary example is crop rotation, where different crop species are planted in succession in each location from season to season. Over multiple years, the same field is planted with a diversity of crops, although the crops may be grown one at a time or in polyculture. Cover cropping, the practice of planting an additional crop between seasons or during fallow years, adds temporal diversity. Although they are not intended for harvesting, cover crops can add C to the soil by providing additional root biomass while also building soil structure and protecting against erosion. Living soil cover, which includes cover crops and perennials (i.e., plants that grow for multiple years, in contrast to annuals, which must be replanted each season), maintains live roots that support soil structure year-round. As discussed in the previous section, nonliving cover such as crop residues is another source of soil organic matter (SOM) that can improve nutrient and water retention and support microbial activity.
Crops strategically integrated into a diverse agricultural system have complementary functions, with one of the most important functions being nitrogen fixation. In a unique partnership, legumes such as groundnuts (peanuts) and beans form root nodules that house nitrogen-fixing bacteria and feed the bacteria carbohydrates while the bacteria convert nitrogen (N) gas into a mineral form that plants can use. Excess N not removed by harvesting adds to the soil nutrient pool that can be mineralized for uptake by future plant roots and other soil organisms. This service of replenishing soil N is so critical that the Conservation Farming Unit in Zambia defines crop diversification as planting 30% of the cultivated area with legumes. The organization promotes the practice as one of the three pillars of conservation agriculture (Conservation Farming Unit, 2017). See the vignette for examples of indigenous crops with benefits in cultivating marginal agroecosystems.
Agricultural biodiversity includes animals as well as plants. In addition to providing harvests that feed humans directly, crops leave residues commonly used as animal feed (although, as subsequently discussed, this presents a tradeoff with maintaining SOM). In many parts of the world, the production of plant products also benefits significantly from animal traction. Milk and meat products provide nutritious food and a source of farm income, while waste products such as animal manure can be added back to the soil to recycle nutrients and build SOM. An organic amendment, manure contributes to the soil’s functional capacity as a reservoir of plant nutrients, a buffer for acidity, and a water supplier. Managing biodiversity within an agroecosystem requires consideration of the full nutrient cycle, tracing nutrients that move from soil to plant to animal and facilitating their return to the soil.
Agroecosystems: Non-crop species can protect or threaten crop yields.
Not all forms of biodiversity in an agroecosystem are useful. Overgrown weeds can deplete the soil of plant-available nutrients; out-compete crop plants for sunlight, space, or water; and inhibit overall crop growth. Mechanical weed management can be time-consuming, especially in conservation agriculture systems where weeds are not uprooted by tillage. Organic weed management strategies include browsing by livestock and growing weed-suppressing cover crops. Where they are accessible, herbicides can be helpful, but their use requires caution to protect crop plants and limit the evolution and growth of resistant weeds.
Another threat to crop production is pests, including insects, arthropods, small mammals, and other animals that damage plants, seeds, or fruits. Dependence on purchased pesticides can be problematic and dangerous for resource-poor farmers. They may not be able to afford sufficient personal protective equipment and suffer adverse health impacts from pesticide application. Training on proper pesticide use might not be accessible, and the financial investment of buying pesticides upfront may not pay off every year. Similar to herbicides, pesticides must be used with caution to prevent the emergence of resistant pests.
Additionally, pesticides may harm beneficial insects or the natural predators of pests. Integrated pest management involves the strategic, limited use of pesticides alongside multiple other tactics to manage pests. One such tactic is biological control or the introduction or enhancement of natural enemy populations (predators or parasites). Other alternative strategies include intercropping with a non-host crop that will repel a pest and using an attractive plant to lure pests away from the crop. Combined, these two strategies are known as a “push–pull” pest management system. To learn more about the implementation of integrated pest management in sub-Saharan Africa, see Van Huis (2009).
Diseases caused by viruses, bacteria, and other microorganisms are another threat to agricultural yields. Some crop diseases can also harm the health of product consumers. For example, aflatoxin produced by fungi in the genus Aspergillus can cause stunted growth, cancer, and other illnesses in people and animals that consume contaminated maize and other cereals (Bandyopadhyay et al., 2007). Scientists are studying plant microbiomes to understand better how to maintain a healthy microbial community and even how to design microbial inoculants that can protect plants from pathogens (Vannier et al., 2019). In animal agriculture, antibiotics can be used to prevent and treat some diseases, but the emergence of antibiotic-resistant strains is an ever-increasing threat to both animal and human health (Manyi-Loh et al., 2018). Twenty-first-century advances in high-throughput molecular analysis technologies such as genetic sequencing and metabolomic analysis provide scientists with many tools to help farmers better understand and manage agricultural microbiomes.
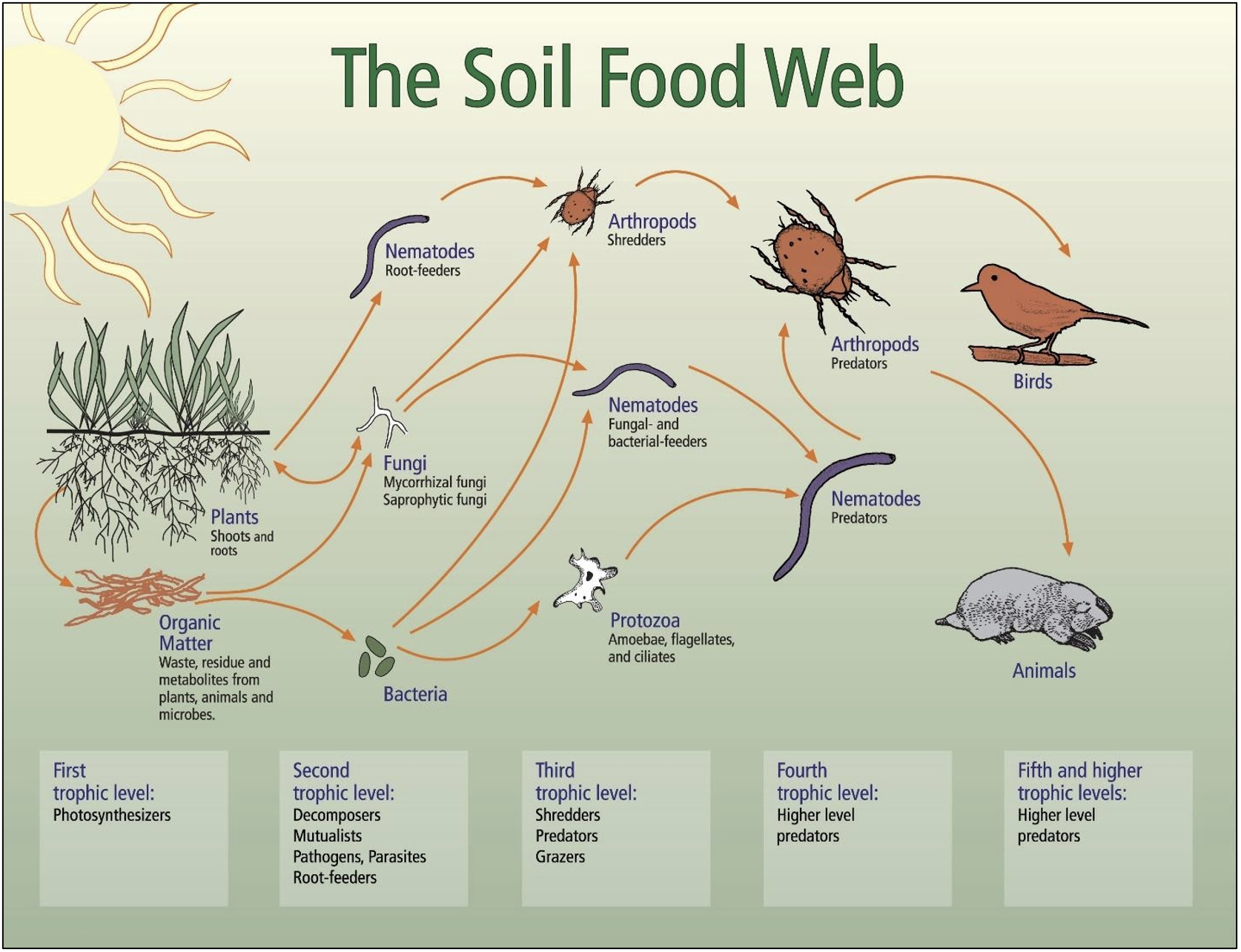
Agroecosystems: Soil contains nutrient reservoirs that support microbe, plant, and animal life.
Soil is a dynamic ecosystem, with micro- and macro-organisms inhabiting a complex of water- and air-filled pores formed by a matrix of mineral and organic particles. Refer to Chapter 3 if you wish to review how soil supports agriculture by providing a medium for plant growth and supplying nutrients to roots. In this chapter, we explore the role of soil organisms in agricultural systems (Table 1).
Table 1
Type of Soil Organisms | Major Functions |
---|---|
Photosynthesizers:
|
Capture Energy:
|
Decomposers:
|
Break down residue
|
Mutualists
|
Enhance Plant Growth
|
Pathogens and Parasites
|
Promote Disease
|
Root-feeders
|
Consume plant roots
|
Bacterial Feeders
|
Graze
|
Fungal Feeders
|
Graze
|
Shredders
|
Break down residue and enhance soil structure
|
Higher-level predators
|
Control populations
|
At the microscopic level, soil microorganisms such as bacteria and fungi are key players in maintaining a healthy soil ecosystem for crop growth. Although soil microbes provide many services to support crop growth, three important ones are building soil structure, decomposing organic matter (OM), and aiding roots in nutrient acquisition. Fungal filaments and bacterial exudates build soil structure by binding together mineral particles to form a matrix of pores and aggregates (see image). OM decomposition occurs when soil microorganisms break down crop residues, manure, or other OM at the molecular level. Ultimately, this process allows the release of mineral nutrients in forms that plant roots can absorb. As for aiding root nutrient acquisition, some plants and microorganisms are symbiotic. For example, the mutually beneficial partnership between legume roots and N-fixing bacteria was described earlier in this chapter. While not all plants form a specific partnership with soil microorganisms in this way, they all can benefit from the nutrients released by microbial OM decomposition and a stable soil structure held together by microbial filaments and exudates.
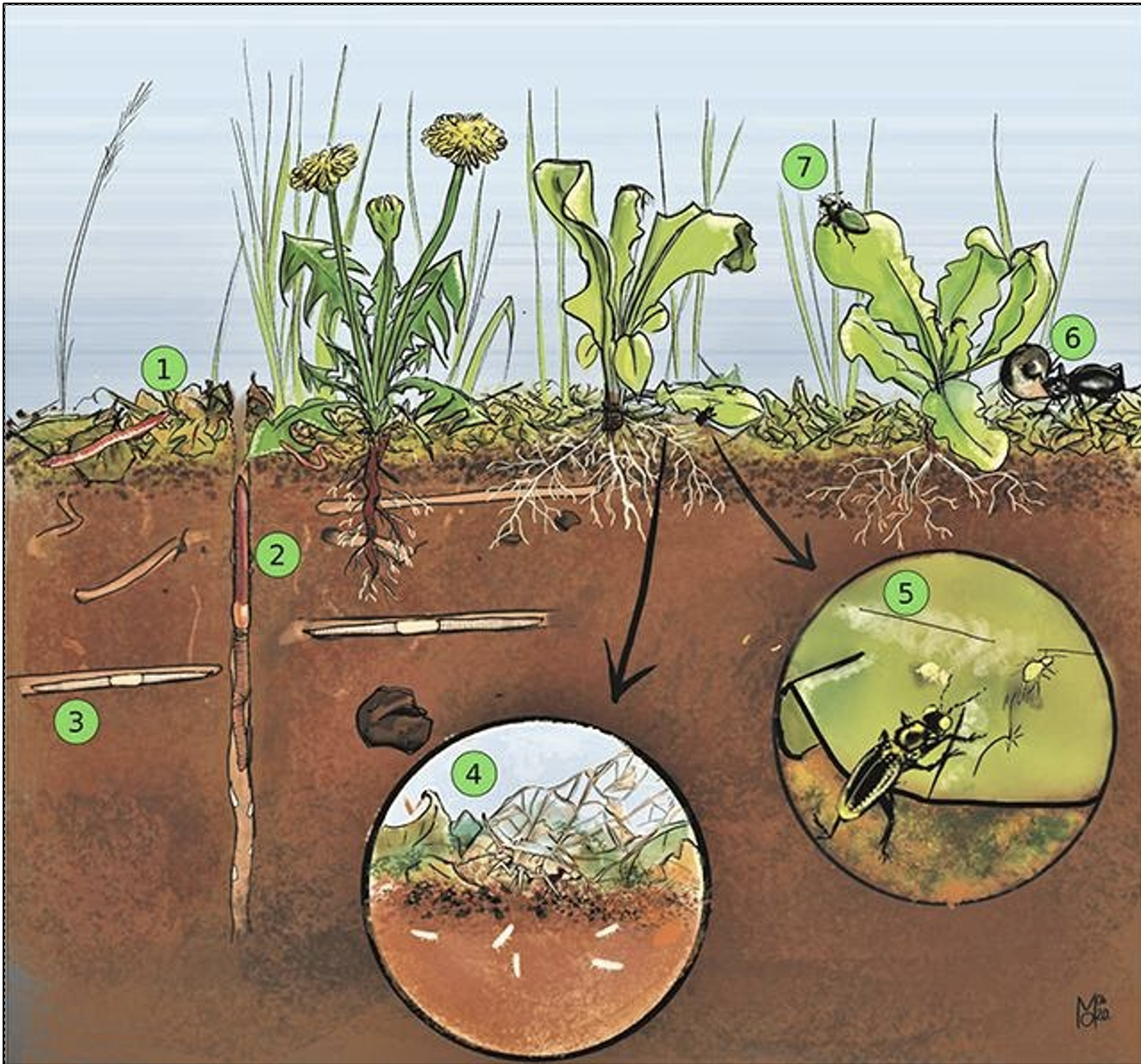
Soil microorganisms are related to other soil life through the soil food web (see image). Moving up the food chain, protozoans graze on bacteria, which releases excess nutrients for uptake by plants and other organisms. Soil invertebrates, including earthworms and arthropods, incorporate OM from the surface deeper into the soil profile and shred it into smaller pieces, allowing microorganisms greater access to it. Some organisms are termed ecosystem engineers due to their burrowing activity that creates macropores in the soil, which in turn allow water and air to penetrate and reach plant roots easily. An example of termites as soil ecosystem engineers in agriculture is provided in this video of an experienced farmer combating deforestation in Burkina Faso.
Soil biodiversity can be positively or negatively impacted by management practices in agricultural systems, especially the application of amendments and the use of tillage. Soil amendments are materials added to the soil by farmers, such as fertilizers and composts. Organic, carbon-rich amendments can increase SOM content, providing energy and nutrients that fuel the soil food web (Masvaya et al., 2017). Manure as an agricultural amendment is further discussed in the following section. Inorganic mineral fertilizers can stimulate soil biological activity; however, without carbon inputs, SOM will ultimately be depleted, limiting soil organisms’ energy sources and harming soil physical quality. Intensive tillage can also deplete SOM because it quickly incorporates surface OM and oxygen into the soil, speeding up decomposition.
Tillage can also destroy soil structure by disrupting microbial filaments and killing soil invertebrates. In contrast, reduced or no tillage has the opposite effect: it preserves SOM content and structure. Additionally, retaining crop residues on the surface protects soil structure from destruction via rain and erosion and improves soil water retention. Compared with burning or removing residues, leaving residues in the field provides another source of SOM.
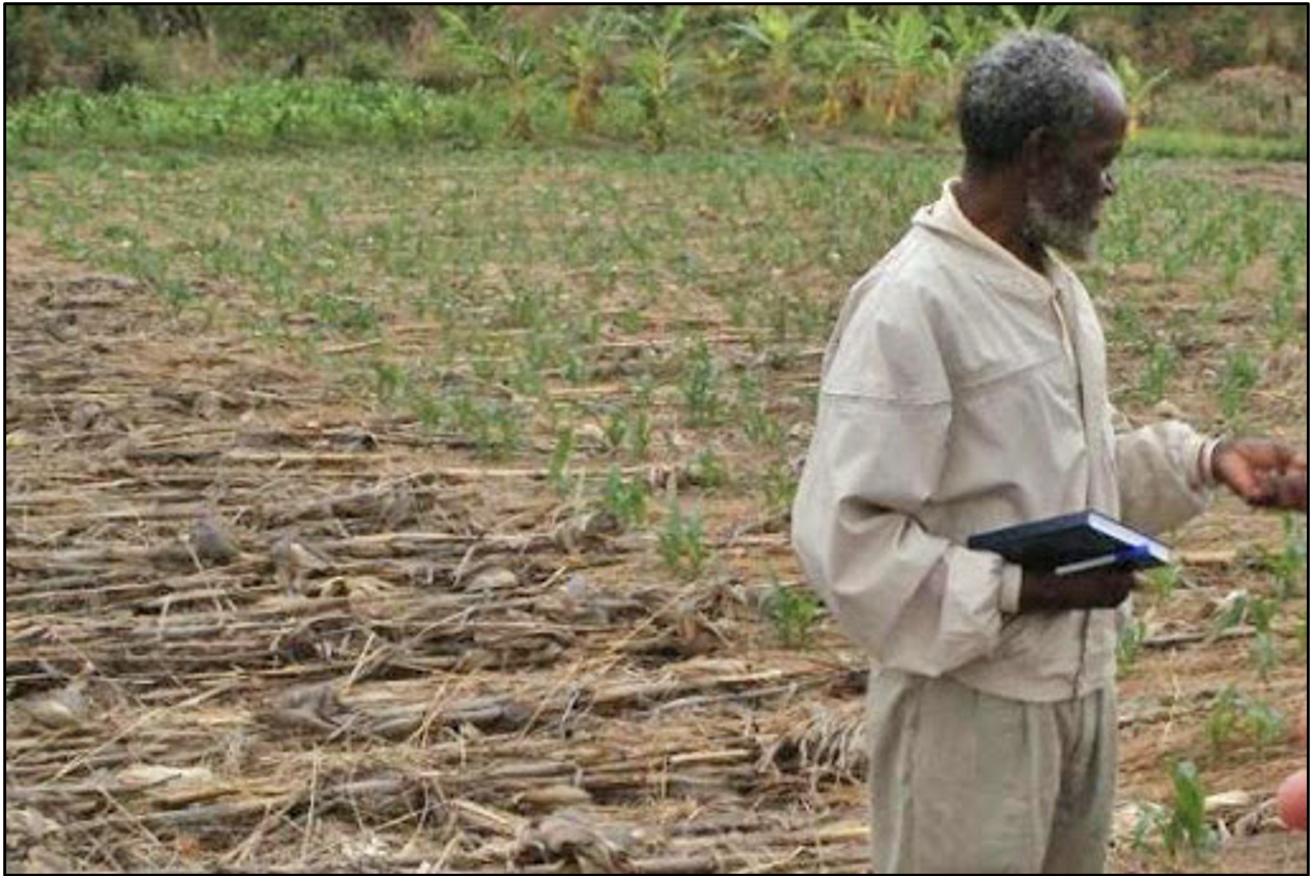
Let’s consider some examples of conservation agriculture (CA) practices in southern Africa that protect soil health and biodiversity. In Zambia, the Conservation Farming Unit (2017) defines minimum soil disturbance as planting in shallow basins or narrow rip lines on flat fields rather than in raised ridges completely tilled each year, and soil cover means leaving crop residues on the ground rather than burning them. (In addition to considering minimal soil disturbance and continuous soil cover, we have already discussed the third pillar of CA in this chapter: crop diversity.) A synthesis of several studies across Malawi, Zimbabwe, and Zambia reported that 80% of site-specific comparisons between conservation and conventional cropping systems showed higher maize yields under CA (Thierfelder et al., 2015). Some central mechanisms to which yield gains experienced under CA are attributed include improved soil health, mitigation of water-related risks, management of biodiversity, and earlier planting times. In on-station trials in Zambia, CA practices have been found to mitigate the loss in total soil carbon observed over time in conventional maize farming (Thierfelder et al., 2013). Similarly, the soil health measures of OM content and aggregate stability are higher under CA residue retention in research plots in Zambia (Thierfelder & Wall, 2010). These examples of reduced tillage and residue retention as two core pillars of CA illustrate CA’s benefits for SOM, the soil food web, and agricultural yields.
Biodiversity Conservation and Food Security
Dr. Tafadzwanashe Mabhaudhi
The conservation of biodiversity in agriculture is important for food security in semi-arid environments. Biodiversity in this context refers to the diversity of food sources and food systems. Indigenous systems benefit food security by increasing the availability, accessibility, utilization, and stability of food.
Traditional and diversified cropping systems can increase food availability. Compared to mono-cropped systems, traditional systems increase the number of food sources. Because indigenous crops are native to the local environment and have minimal growing requirements, their production costs, both to farmers and to the environment, are minimal. This improves accessibility. In most cases, indigenous crops are landraces, which have seeds that farmers can exchange, maintain, and retain for themselves across seasons. This also improves accessibility.
Utilization is where the challenge is. In some cases, indigenous knowledge systems have been eroded, and there is a perception that these crops are “poor man’s crops.” This creates a cultural hurdle for the utilization of indigenous species. In addition to developing interventions aimed at making indigenous crops available and accessible, it is necessary to take a holistic approach to encourage utilization. To do so, we consider the palatability of substituting indigenous crops in different types of dishes that are socially and culturally acceptable.
The stability aspect of food security is linked to climate change resilience, or the ability of the system to bounce back after an environmental shock. High-input, high-output systems tend to have a high sensitivity to external shock, while low input systems tend to be stable over a longer period.
In the context of Southern Africa, climate change’s main impacts are rising temperatures and increasing water scarcity. Our research confirms that many indigenous crops are more tolerant of drought and heat stress. They therefore have many benefits for future environments that may be hotter and drier due to climate change. We have done modeling work through the year 2100 to compare how different crops will behave. In the case of Bambara nut, we found that areas suitable for its cultivation are predicted to increase in the future along with its yields and water use efficiency. In contrast, areas suitable for major crops like maize and wheat are predicted to shrink.
Biodiversity Conservation and Agroecosystem Boundaries
Forest Conservation for Food Security
Dr. Tafadzwanashe Mabhaudhi
In our current projects in South Africa, forests are viewed as a source of food. Early studies that we conducted showed that wild foods in the understory of forests (e.g., (wild fruits, mushrooms, honey, leafy greens, insects, and animals) were important sources of nutrition. In the old days, foraging was a regular part of South African culture. Over time in colonial history, people were forcibly resettled into areas where forests were not available. In addition, many neighboring forests were managed as a commercial operation where local populations were not allowed to forage. Fire management concerns caused timber companies to restrict people from using forest products. As a result, local populations were restricted from using culturally relevant agroforestry practices, such as planting traditional crops in the understory of forests. In our current project, we are exploring traditional designs in updated forms to improve food security.
Biodiversity conservation through agroecological principles does not end at the boundaries of the farm. Land conversion from natural forest to agriculture is a major contributor to biodiversity loss. Take South Africa, for example. Due to the pressures of overharvesting and land clearing for agriculture, many regions in South Africa experience concerns about the ability of tropical woodlands to sustain the services that are essential to the subsistence of local populations (Wessels et al., 2011). Historically, a major conservation focus of governments and nonprofit environmental groups has been the establishment of protected areas where human activity is minimized or restricted (Constant & Taylor, 2020). This can be a crucial strategy when protecting endangered species or threatened habitats. It also generates income through ecotourism.
Although protected areas like national parks and game reserves are important for wildlife conservation, restricting access to forests has not slowed rates of deforestation in South Africa. People need forests for survival. In chronically food-insecure regions, woodland ecosystems provide several essential services. Forests provision forage for livestock grazing, timber and firewood, and wild foods like fruits, mushrooms, honey, leafy greens, insects, and animals. Forests and savannahs are valuable resources for chronically food-insecure populations (Constant & Taylor, 2020).
Forest Conservation for Food Security
Dr. Tafadzwanashe Mabhaudhi
Conservation needs to change how people relate to the environment. In the past, reforestation and biodiversity conservation efforts were met with resistance from local populations. These conservation efforts were very protectionist, forcing people to stay out of forests rather than educating the population about the value of forest resources. Approaches to conservation are beginning to change by considering local land uses and indigenous values. If communities valued forests and feel a sense of ownership over the resource, they would cultivate a sense of cultural pride, dignity, and social cohesion regarding forest management. Therefore, we are looking at reforestation and forest conservation from a food security perspective. If food security is the major issue in the region, then reforestation must be framed around this issue. People will not relate to reforestation efforts if reforestation doesn’t meet their most important needs.
Access to forest provisioning and cultural services are key drivers of biodiversity conservation. Neglecting the human element of forest conservation can alter the flow of cultural services that forests provide, leading to the loss of identity and knowledge systems, social conflicts, and diminished trust in institutions (Constant & Taylor, 2020; Lele et al., 2010). Rather than simply restricting access to forests, new conservation efforts consider multiple strategies for forest rehabilitation. The goal is to create spaces for natural regeneration or active restoration at the boundaries of agricultural land, including in environmentally important areas, marginal landscapes, farm forestry, and agroforestry systems (Constant & Taylor, 2020). In these cases, including all stakeholder perspectives is essential to inform environmental decisionmaking. Increased engagement of indigenous and local people and their knowledge of ecosystem dynamics can inform the restoration of forest ecosystem services. Farmers are valuable in conservation efforts. They have intimate knowledge of the resource base and can inform policymakers regarding site selection and land management regimes (Constant & Taylor, 2020).
Check Your Knowledge
- What are agroecosystems? How does agriculture affect ecosystem services?
- In what ways can non-harvested species in agricultural systems benefit crop growth? Think about soil organisms, cover crops, and natural predators, among others.
- What agricultural management practices might have a negative impact on biodiversity? For each practice, consider an alternative. What are the potential risks and benefits associated with it?
- Compare and contrast a monocultural system with a diverse cropping system. What are the challenges of each system?
- If improved seeds are developed to grow high-yielding varieties, what is the value of landraces?
- How are human cultural values considered in biodiversity conservation efforts?
- What specific questions would you ask a farmer to learn about how biodiversity is managed on their farm?
References
Altieri, M. A. (2002). Agroecology: The science of natural resource management for poor farmers in marginal environments. Agriculture, Ecosystems & Environment, 93(1–3), 1–24.
Ariga, J., Mabaya, E., Waithaka, M., & Wanzala‐Mlobela, M. (2019). Can improved agricultural technologies spur a green revolution in Africa? A multicountry analysis of seed and fertilizer delivery systems. Agricultural Economics, 50(S1), 63–74. https://doi.org/10.1111/agec.12533
Bandyopadhyay, R., Kumar, M., & Leslie, J. F. (2007). Relative severity of aflatoxin contamination of cereal crops in West Africa. Food Additives and Contaminants, 24(10), 1109–1114. https://doi.org/10.1080/02652030701553251
Chain-Guadarrama, A., Martínez-Salinas, A., Aristizábal, N., & Ricketts, T. H. (2019). Ecosystem services by birds and bees to coffee in a changing climate: A review of coffee berry borer control and pollination. Agriculture, Ecosystems & Environment, 280, 53–67. https://doi.org/10.1016/j.agee.2019.04.011
Conservation Farming Unit. (2017). Conservation farming and climate smart agriculture for farmers in agro-ecological regions I & IIa Zambia: A handbook for field technicians and lead farmers. Conservation Farming Unit.
Constant, N. L., & Taylor, P. J. (2020). Restoring the forest revives our culture: Ecosystem services and values for ecological restoration across the rural-urban nexus in South Africa. Forest Policy and Economics, 118, Article 102222.
Daryanto, S., Fu, B., Zhao, W., Wang, S., Jacinthe, P. A., & Wang, L. (2020). Ecosystem service provision of grain legume and cereal intercropping in Africa. Agricultural Systems, 178, Article 102761. https://doi.org/10.1016/j.agsy.2019.102761
Dwivedi, S. L., Ceccarelli, S., Blair, M. W., Upadhyaya, H. D., Are, A. K., & Ortiz, R. (2016). Landrace germplasm for improving yield and abiotic stress adaptation. Trends in Plant Science, 21(1), 31–42. https://doi.org/10.1016/j.tplants.2015.10.012
Gaston, K. J., & Spicer, J. I. (2013). Biodiversity: An introduction. John Wiley & Sons.
Galluzzi, G., Van Duijvendijk, C., Collette, L., Azzu, N., & Hodgkin, T. (Eds.). (2011). Biodiversity for food and agriculture: Contributing to food security and sustainability in a changing world. Food and Agriculture Organization of the United Nations and Platform for Agrobiodiversity Research. http://www.fao.org/fileadmin/templates/biodiversity_paia/PAR-FAO-book_lr.pdf
Leemans, R., & De Groot, R. S. (2003). Ecosystems and human well-being: A framework for assessment. Island Press.
Lele, S., Wilshusen, P., Brockington, D., Seidler, R., & Bawa, K. (2010). Beyond exclusion: Alternative approaches to biodiversity conservation in the developing tropics. Current Opinion in Environmental Sustainability, 2(1–2), 94–100.
Malézieux, E., Crozat, Y., Dupraz, C., Laurans, M., Makowski, D., Ozier-Lafontaine, H., Rapidel, B., De Tourdonnet, S., & Valantin-Morison, M. (2009). Mixing plant species in cropping systems: Concepts, tools and models: A review. In E. Lichtfouse, M. Navarrete, P. Debaeke, S. Véronique, & C. Alberola (Eds.), Sustainable Agriculture (pp. 329–353). Springer, Dordrecht. https://doi.org/10.1007/978-90-481-2666-8_22
Manyi-Loh, C., Mamphweli, S., Meyer, E., & Okoh, A. (2018). Antibiotic use in agriculture and its consequential resistance in environmental sources: Potential public health implications. Molecules, 23(4). MDPI AG. https://doi.org/10.3390/molecules23040795
Masvaya, E. N., Nyamangara, J., Descheemaeker, K., & Giller, K. E. (2017). Tillage, mulch and fertiliser impacts on soil nitrogen availability and maize production in semi-arid Zimbabwe. Soil and Tillage Research, 168, 125–132. https://doi.org/10.1016/j.still.2016.12.007
Pretty, J., Toulmin, C., & Williams, S. (2011). Sustainable intensification in African agriculture. International Journal of Agricultural Sustainability, 9(1), 5–24.
Thierfelder, C., Matemba-Mutasa, R., & Rusinamhodzi, L. (2015). Yield response of maize (Zea mays L.) to conservation agriculture cropping system in Southern Africa. Soil and Tillage Research, 146(PB), 230–242. https://doi.org/10.1016/j.still.2014.10.015
Thierfelder, C., Mwila, M., & Rusinamhodzi, L. (2013). Conservation agriculture in eastern and southern provinces of Zambia: Long-term effects on soil quality and maize productivity. Soil and Tillage Research, 126, 246–258. https://doi.org/10.1016/j.still.2012.09.002
Thierfelder, C., & Wall, P. C. (2010). Rotation in conservation agriculture systems of Zambia: Effects on soil quality and water relations. Experimental Agriculture, 46(3), 309–325. https://doi.org/10.1017/S001447971000030X
Van Huis, A. (2009). Challenges of integrated pest management in sub-Saharan Africa. Integrated Pest Management, 2, 395–417). Springer Netherlands. https://doi.org/10.1007/978-1-4020-8990-9_12
Vannier, N., Agler, M., & Hacquard, S. (2019). Microbiota-mediated disease resistance in plants. PLoS Pathogens, 15(6). https://doi.org/10.1371/journal.ppat.1007740
Wessels, K. J., Mathieu, R., Erasmus, B. F. N., Asner, G. P., Smit, I. P. J., Van Aardt, J. A. N., & Jacobson, J. (2011). Impact of communal land use and conservation on woody vegetation structure in the Lowveld savannas of South Africa. Forest Ecology and Management, 261(1), 19–29.
Zeven, A. C. (1998). Landraces: A review of definitions and classifications. Euphytica, 104, 127–139.